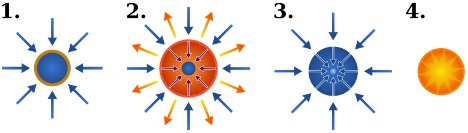
Figure 1: The image above shows the steps for inertial confinement fusion. In the first step, energy is concentrated toward a target with the materials for fusion in the center, after which point, in step two, the energy causes an explosion, which creates compression. In the third step, compression generates the extreme thermal conditions needed for nuclear fusion, and then in the fourth step, nuclear fusion occurs
Source: Wikimedia Commons
As global warming continues to threaten the world, billions of dollars have been poured into researching alternative, climate-friendly energy sources. One of the most anticipated of these alternative energy sources is nuclear fusion (not to be confused with nuclear fission). In nuclear fusion, two atoms merge to form a single heavy nucleus, and the leftover mass is translated into a large amount of energy, quantified by Einstein’s equation of E = mc2 (Energy.gov, 2020). On Earth, this reaction is most easily achieved through two hydrogen isotopes, deuterium and tritium, since they are able to fuse at lower temperatures relative to other atoms. Deuterium and tritium combine in a fusion reaction to form a helium atom with an extra neutron and leftover mass. What makes nuclear fusion enticing for scientists is that deuterium and tritium are readily found in ordinary seawater, meaning that, so long as there is water, there will be sufficient fuel for fusion (American Nuclear Society, 2013). Additionally, this reaction does not release any harmful waste that might negatively impact the environment. Given the advantages, however, achieving stable, sustainable nuclear fusion is also accompanied by its own set of challenges.
For fusion to occur, the nuclei of the two hydrogen isotopes need to overcome their electrostatic forces that push them apart, but this is only possible in extreme environments that are extremely dense and have temperatures around 50 million degrees celsius. Under such conditions, electrons are stripped from atomic nuclei to form plasma or ionized gas, and the atoms are no longer subject to the electrostatic force holding them apart (American Nuclear Society, 2013). These environments are not completely theoretical – these conditions present themselves in situations where immense gravity and high temperatures can overcome the repulsive force, such as stars (including our Sun), but it is difficult to replicate these conditions given the constraints on Earth.
The most thoroughly researched method of achieving nuclear fusion is known as ‘magnetic confinement.’ This approach uses powerful magnets to trap and squeeze atoms, increasing density and heating them to temperatures ten times the Sun’s core (Michaels, 2020). Although possibly effective, this method has many drawbacks, including the cost of building this type of reactor and mechanical problems. It also takes more energy to stimulate magnetic confinement than fusion releases, meaning this method will not be financially viable. For these reasons, an England-based company named ‘First Light Fusion’ is exploring another technique to achieve fusion, and their inspiration came from an unlikely source: the pistol shrimp.
The pistol shrimp is a small creature, around 4 centimeters in length, yet has the remarkable ability to stun its prey with a water jet. To do this, the pistol shrimp moves its claws at speeds close to 1.6 kilometers per second, and this speed is so great that the internal pressure of the water lowers, and bubbles consisting of a vacuum start to form (Nag, 2017). As the pressure builds back up, the bubbles burst, and the force from the explosion of the bubble causes extreme compression, instantly generating enormous heat which can then be funneled towards its prey to stun them. This entire explosion and compression phenomenon is called ‘inertial confinement,’ and the only other known naturally occurring instance of this phenomenon is a supernova (Nag, 2017). First Light Fusion’s goal is to artificially recreate the inertial confinement phenomenon to create the conditions necesssary for nuclear fusion.
To this end, the company created a small target, called a ‘pellet,’ containing deuterium and tritium. Then, to achieve inertial confinement, they focus an enormous amount of energy on the tiny pellet in hopes that, when they focus enough energy, the outer shell will explode while compressing the center long enough for the conditions for fusion to build up. Eventually, this will cause the deuterium and tritium to combine (Wade, 2018). Other than the ‘pellet,’ which is patented by the company, the true innovation comes from the system that delivers the energy onto the pellet. The established method for focusing energy onto a pellet the use of a high-powered laser, employed by the National Ignition Facility. However, despite the established method, First Light Fusion elected to take a different approach. They developed a high-voltage pulse power device that uses electromagnetism to fire hypervelocity projectiles at speeds of 20 kilometers per second (Wade, 2018). This device, named ‘Machine 3,’ is capable of focusing 13.3 mega Amps, comparable to 500 lightning strikes, onto a single target. According to Nicholas Hawker, co-founder of First Light Fusion, “the cost per joule of energy is one of the most critical elements for fusion and using Machine 3 to launch the projectile is 1,000 times cheaper, per joule of energy, than using a laser” (Wade, 2018).
The next step for the company is to demonstrate energy gain from nuclear fusion. To reach this goal, they have begun construction on Machine 4, which they anticipate will be able to shoot its hypervelocity projectiles at a target once every five seconds (Wade, 2018). If this small startup can finally make nuclear fusion financially viable, it could have wide-ranging implications for all aspects of human life. They could finally end the war against climate change by developing a crucial weapon that will render our deep reliance on poisonous fossil fuels obsolete.
References
Energy.gov. (2020, September 7). DOE Explains…Nuclear Fusion Reactions. Retrieved January 17, 2021, from: https://www.energy.gov/science/doe-explainsnuclear-fusion-reactions#:~:text=Nuclear%20Fusion%20reactions%20power%20the,The%20leftover%20mass%20becomes%20energy.
American Nuclear Society. (2013, October 13). Nuclear Fusion. Retrieved January 17, 2021, from: http://nuclearconnect.org/know-nuclear/science/nuclear-fusion.
Michaels, D. (2020, February 06). Fusion Startups Step In to Realize Decades-Old Clean Power Dream. Retrieved January 17, 2021, from: https://www.wsj.com/articles/fusion-startups-step-in-to-realize-decades-old-clean-power-dream-11581001383?&mod=djemfoe.
Nag, R. (2017, December 11). The Energy Physics of the Pistol Shrimp. Retrieved January 17, 2021, from: http://large.stanford.edu/courses/2017/ph240/nag2/.
Wade, A. (2018, May 17). How the pistol shrimp prompted an attempt at energy gain from fusion. Retrieved January 17, 2021, from: https://www.theengineer.co.uk/energy-gain-fusion/.
Related Posts
Speed of Light Reduced Using Nanoantennas
Figure 1: The speed of light is extremely fast–186,00 miles...
Read MoreTheoretical Astrophysics Research on How The Big Crunch Builds to the Big Bounce Theory
Cover Figure: Simulated timeline of Hubble expansion since the formation...
Read MoreModeling Maximum Running Speed in Animals
Figure: Two cheetahs lie side by side. As the fastest...
Read MorePalin Narsian