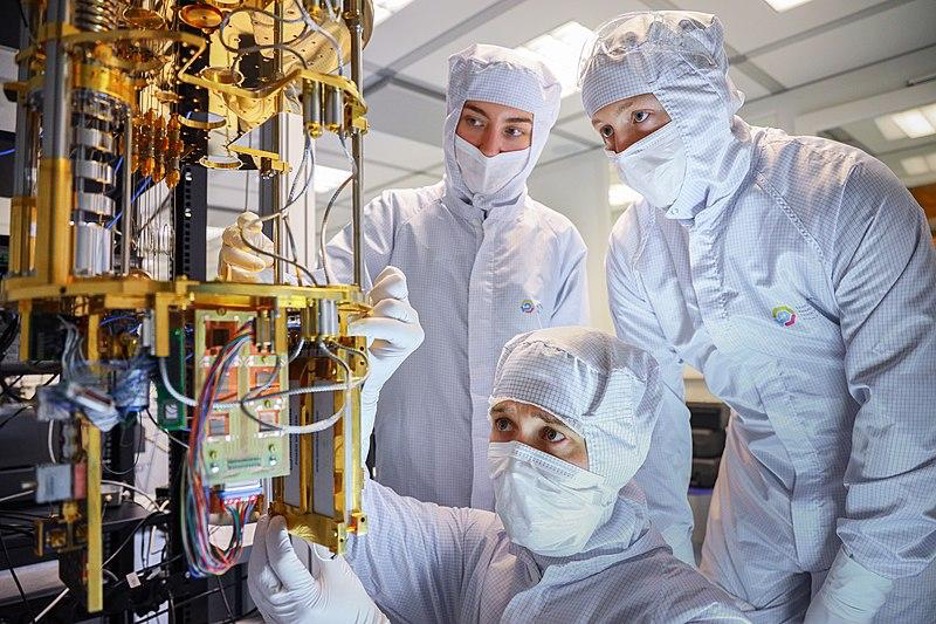
Figure 1: These scientists are assembling a part of a quantum computer. The superconducting qubits, the fundamental units of a quantum computer, need to be temperatures close to absolute zero in order to work, which illuminates the delicacy of them. This fragility does not end, as researchers have found that natural radiation plays a big part in hindering qubit performance.2
Image Citation: Wikimedia Commons
Quantum computers have the potential to surpass the most capable of today’s supercomputers. Quantum computers harness some of the near-mystical phenomena that are explained by quantum mechanics to provide more efficient methods of computing. Although regular computers are still the easiest and most economical solution for solving most problems, quantum computers show promise to power exciting innovations in various fields. Classical computing has long relied on bits, a current of electrical impulses representing 0s and 1s, to contain information in order to carry out calculations, but quantum computing’s success stems from its employment of a quantum bit, or qubit. Qubits, typically represented by subatomic particles such as electrons or photons, are useful because their electrical current of impulses represent values of 0 and 1 simultaneously to different degrees. This ability, called superposition, allows the computer to run multiple potential outcomes of a calculation at the same time. Furthermore, scientists can generate pairs of qubits that are virtually “connected” with one another. This phenomenon, called “entanglement,” dictates that changing the state of one qubit will instantaneously change the qubit that it is “entangled” with (this happens even if they are separated by immense distances). This is useful because in a classical computer doubling the number of bits doubles its computational ability, but with entangled qubits, adding qubits results in an exponential increase in computational power (2n computational power, where n is the number of qubits added). Although this potential is exciting, qubits are much more sensitive and error-prone than regular bits, and a recent study has shown just how sensitive they really are.1
A multidisciplinary research team, consisting of researchers from the U.S. Department of Energy’s Pacific Northwest National Laboratory (PNNL) and the Massachusetts Institute of Technology (MIT), has shown that radiation emitted from natural sources in the environment can limit the performance of qubits. This interference of the quantum state, known as “decoherence,” causes the qubits to be disturbed and “pushed” out of their prepared states, which makes them non-functional. Their study measured the performance of prototype qubits in two different experiments: one in which the qubits were exposed to elevated radiation, and the other in which they built a shield around the qubits to limit the amount of natural radiation. The pair of experiments clearly showed that an increase of radiation directly correlated with a lower length of time that the qubits remained in their coherent state. More specifically, they found that the radiation broke apart pairs of electrons that normally carry the current of electrical impulses between the qubits and enable their functionality. The breaking of these electrons caused resistance in the current that destroyed the delicately prepared and highly sensitive state of the qubit.2
Quantum computers have the potential to surpass the most capable of today’s supercomputers. Quantum computers harness some of the near-mystical phenomena that are explained by quantum mechanics to provide more efficient methods of computing. Although regular computers are still the easiest and most economical solution for solving most problems, quantum computers show promise to power exciting innovations in various fields. Classical computing has long relied on bits, a current of electrical impulses representing 0s and 1s, to contain information in order to carry out calculations, but quantum computing’s success stems from its employment of a quantum bit, or qubit. Qubits, typically represented by subatomic particles such as electrons or photons, are useful because their electrical current of impulses represent values of 0 and 1 simultaneously to different degrees. This ability, called superposition, allows the computer to run multiple potential outcomes of a calculation at the same time. Furthermore, scientists can generate pairs of qubits that are virtually “connected” with one another. This phenomenon, called “entanglement,” dictates that changing the state of one qubit will instantaneously change the qubit that it is “entangled” with (this happens even if they are separated by immense distances). This is useful because in a classical computer doubling the number of bits doubles its computational ability, but with entangled qubits, adding qubits results in an exponential increase in computational power (2n computational power, where n is the number of qubits added). Although this potential is exciting, qubits are much more sensitive and error-prone than regular bits, and a recent study has shown just how sensitive they really are.1
A multidisciplinary research team, consisting of researchers from the U.S. Department of Energy’s Pacific Northwest National Laboratory (PNNL) and the Massachusetts Institute of Technology (MIT), has shown that radiation emitted from natural sources in the environment can limit the performance of qubits. This interference of the quantum state, known as “decoherence,” causes the qubits to be disturbed and “pushed” out of their prepared states, which makes them non-functional. Their study measured the performance of prototype qubits in two different experiments: one in which the qubits were exposed to elevated radiation, and the other in which they built a shield around the qubits to limit the amount of natural radiation. The pair of experiments clearly showed that an increase of radiation directly correlated with a lower length of time that the qubits remained in their coherent state. More specifically, they found that the radiation broke apart pairs of electrons that normally carry the current of electrical impulses between the qubits and enable their functionality. The breaking of these electrons caused resistance in the current that destroyed the delicately prepared and highly sensitive state of the qubit.2
The researchers concluded that the findings have direct implications for qubit design and construction. First, the researchers suggested that the blueprint used to construct quantum computers should omit materials that emit radiation. Additionally, the researchers suggest that further shielding should be implemented to protect the quantum computers from radiation in the atmosphere. To address this, interest has turned to whether PNNL’s Shallow Underground Laboratory (a sixty-foot underground laboratory located in Richland, Washington), which reduces radiation exposure by 99%, should be implemented for further quantum computer development. Although the findings are important, the researchers acknowledge that factors other than radiation exposure are more prominent hindrances to qubit stability. For example, microscopic defects and impurities in the materials used to construct qubits are thought to be the major reasons for the current performance limits of qubits. However, once these limitations are overcome, radiation begins to assert its obstacles and limit the coherence time of qubits to a few milliseconds, which is far too insufficient with practical quantum computing.2
Although the findings mainly explain the role of radiation on qubit instability, the researchers believe that there may be implications for the search of dark matter, which is thought to comprise about 25% of our universe and be responsible for the rotation of galaxies and other large-scale structures in the universe. Picking up dark matter signals relies on superconducting detectors, which are quite similar to qubits in design. This means that dark matter detectors also need to be shielded from external radiation, for it can trigger false recordings. As Ben Loer, a research physicist at PNNL who works in both dark matter detection and qubits, concludes,
“Improving our understanding of this process may lead to improved designs for these superconducting sensors and lead to more sensitive dark matter searches. We may also be able to use our experience with these particle physics sensors to improve future superconducting qubit designs.”2
References
- Giles, M., (2019, January, 19). Explainer: What is a quantum computer? MIT Technology Review. Retrieved August 26, 2020 from https://www.technologyreview.com/2019/01/29/66141/what-is-quantum-computing/
- DOE/Pacific Northwest National Laboratory. (2020, August 26). Natural radiation can interfere with quantum computers: Study shows the need to shield qubits from natural radiation, like cosmic rays from outer space. ScienceDaily. Retrieved August 26, 2020 from www.sciencedaily.com/releases/2020/08/200826110328.htm
- Vepsäläinen, A.P., Karamlou, A.H, Orrell J.L. et al. (2020, August 26). Impact of ionizing radiation on superconducting qubit coherence. Nature. Retrieved August 26, 2020 from https://www.nature.com/articles/s41586-020-2619-8#citeas
Related Posts
Quantum Entanglement and Specific Qubit Segmentation in Computing and Sensory Technology
Cover Image: This image illustrates a qubit’s (quantum bit) generic...
Read MoreUV-Vis Spectroscopy: A Step in the Light Direction
Executive Board Advisor: Nishi Jain Co-Authors: Daniela Galvez-Cepeda, Katherine Faulkner,...
Read MoreThe Speed of Sound Finally Bounded by Fundamental Constants
Figure 1: FA-18 Hornet breaking the sound barrier (7 July...
Read MoreSai Rayasam