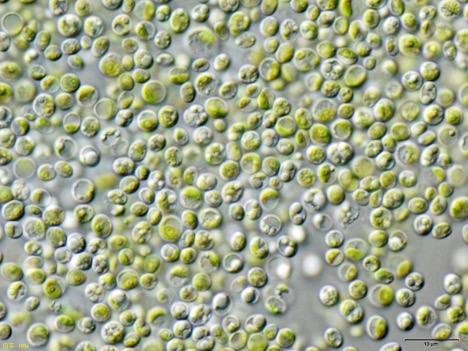
Figure 1: A microscopic image of Chlorella vulgaris, a species of green algae. Green algae have specialized enzymes that can produce clean hydrogen gas from H+ cations under anaerobic conditions. This hydrogen gas could be used as a clean alternative to fossil fuels
Source: Wikimedia Commons
In the quest for developing renewable energy sources, many scientists have investigated the possibility of using molecular hydrogen. Unlike traditional fossil fuels, when hydrogen is subjected to combustion with oxygen the only by-product is water, so hydrogen as a fuel source has the possibility of limiting CO2 emissions. However, gaseous hydrogen is incredibly rare on earth, so it must be produced from other sources, and some processes for hydrogen production (such as coal and biomass gasification) still produce CO2 as a byproduct (Office of Energy Efficiency and Renewable Energy, 2020). Others, such as the electrolysis of water, require large amounts of electrical energy input and rare metals like platinum and iridium for electrodes. This makes hydrogen-based fuel cells prohibitively expensive (Safizadeh et. al, 2015).
Recent studies by Xu et. al have demonstrated an alternative pathway for producing hydrogen: droplet-based “microbial factories” that could produce hydrogen via the action of special hydrogenase enzymes (Xu et. al, 2020). Hydrogenases produce hydrogen by reducing the protons that are a result of the photo-oxidation of water in algal photosynthesis. However, the oxygen produced by photosynthesis typically deactivates hydrogenase activity by binding to the central iron cations and destroying the hydrogen binding site (Stripp et. al, 2009). When a large number of algal cells are packed together, though, sustained hydrogen production from hydrogenases is possible. This is due to the outer “shell” of the algal cell sphere producing H+ cations and electrons from photosynthesis, which the inner “core” turns to gaseous hydrogen under more anaerobic conditions (Xiong et. al, 2015).
To develop these “factories,” the group captured large numbers of Chlorella algal cells in droplets composed of polyethylene glycol and dextran. When placed in a more concentrated polyethylene glycol solution, the droplets experienced hyperosmotic pressure, compacting an average of 9000 cells into micro-droplets that were up to 170 μm in diameter (Xu et. al, 2020). The group found that exposing medium-sized droplets – around 92 μm in diameter – to photosynthetic conditions led to a net drop in dissolved oxygen concentration. This indicated that respiration and hydrogenase activity became dominant over photosynthesis in the core of the droplets (Xu et. al, 2020). They also found that one droplet was capable of producing 1.5 μmol of hydrogen after 96 hours. As some 250,000 droplets could be produced in 1 mL of water, some 0.375 mol of hydrogen could be generated from just 1 mL of this solution (University of Bristol, 2020). Comparatively, hydrogen production by electrolysis takes 0.75 moles, or about 13.5 mL of water to generate the same quantity of hydrogen, but can generate this amount in minutes (Lyle, 2020).
The group also decided to create a larger oxygen-deprived core by coating the droplets with a thin shell of E. Coli. To ensure that the E. Coli remained on the outside of the droplets, they attached polyethylene glycol groups to the outer cell wall to make them more soluble in the external polyethylene glycol solution compared to Chlorella (Xu et. al, 2020). Under these conditions, the hybrid droplets produced 2 μmol of hydrogen in 72 hours, compared to the 1 μmol for the pure Chlorella droplets. They also found this rate of hydrogen production to be 2.2 times the natural rate of hydrogen production (Xu et. al, 2020). Xin Huang, co-author on the published paper, noted that this method did not impede the viability of the cells, suggesting that the droplet systems could be used over longer periods of time. He also said that this system could be used for the production of other compounds; for instance, he noted that the group used a similar system with yeast cells to produce ethanol (University of Bristol, 2020). Ultimately, this droplet method for producing “green” hydrogen could present another, more inexpensive option for creating renewable energy.
References
Lyle, K. (n.d.). Retrieved December 17, 2020, from https://www.chem.purdue.edu/gchelp/howtosolveit/Electrochem/Electrolysis.htm
Office of Energy Efficiency and Renewable Energy. (2020). Hydrogen Fuel Basics. Retrieved December 12, 2020, from https://www.energy.gov/eere/fuelcells/hydrogen fuel-basics
Safizadeh, F., Ghali, E., & Houlachi, G. (2015). Electrocatalysis developments for hydrogen evolution reaction in alkaline solutions – A Review.International Journal of Hydrogen Energy, 40(1), 256-274. doi:10.1016/j.ijhydene.2014.10.109
Stripp, S. T., Goldet, G., Brandmayr, C., Sanganas, O., Vincent, K. A., Haumann, M., . . . Happe, T. (2009). How oxygen attacks [FeFe] hydrogenases from photosynthetic organisms.Proceedings of the National Academy of Sciences,106(41), 17331-17336. doi:10.1073/pnas.0905343106
University of Bristol. (2020, November 25). Research creates hydrogen-producing living droplets, paving way for alternative future energy source. Retrieved December 12, 2020, from https://phys.org/news/2020-11-hydrogen-producing-droplets-paving-alternative-future.html
Xiong, W., Zhao, X., Zhu, G., Shao, C., Li, Y., Ma, W., . . . Tang, R. (2015). Silicification-Induced Cell Aggregation for the Sustainable Production of H2under Aerobic Conditions.Angewandte Chemie International Edition, 54(41), 11961-11965. doi:10.1002/anie.201504634
Related Posts
PCR: Captivation Through Replication
Executive Board Advisor: Nishi Jain Co-Authors: Vivek Babu, Collins Kariuki,...
Read MoreNew-clear Reactors: Newly approved nuclear reactor designs seek to improve on old ones
Figure 1: Shown by the red line, the sharp increase...
Read MoreA New Tool in The Box: Radicals and Organic Synthesis
Figure 1: This shows the resonance structures for a Ketyl...
Read MoreAndrew Sasser
Comments are closed.