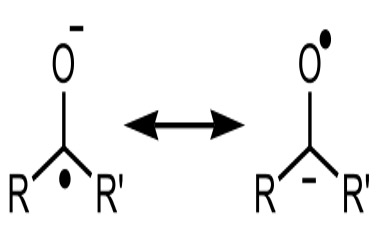
Figure 1: This shows the resonance structures for a Ketyl radical. Due to the presence of an oxygen atom, this radical is significantly more stable due to resonance. Resonance-stabilized radicals have been suggested as efficient catalysts in the synthesis of complex organic products and medicines
Source: Wikimedia Commons
For modern synthetic strategies in the production of complex of many organic molecules, a number of factors play a role in a reaction’s viability for commercial and medicinal use. These methods include measuring the toxicity of involved chemicals, cost of chemical feedstocks, energy inputs, and reaction selectivity. One of the most promising developments in the efficacy of organic synthesis schemes is the use of 1-electron radicals to facilitate carbon-carbon bond formation. Because they only have one electron, these free radicals are typically highly reactive, and act by reducing the energy requirement needed to break and form a variety of bonds (Walling, 2020). Similarly, as Ohio State professor David Nagib described these radicals in a Dartmouth Chemistry department colloquium last Thursday, radicals are commonly believed to be incompatible with organic synthesis due to their tendency to form undesired side products and reduce yield needed to generate them and a believed lack of selectivity. Professor Nagib instead demonstrated the capability of highly-substituted radicals – radicals centered on atoms that are bonded to up to one hydrogen atom – as efficient and highly selective catalysts through polarity reversal and site selective activation, providing a potential new avenue for synthetic strategies (Nagib, 2020). According to Griller and Ingold, these more substituted radicals are more stable due to the potential for resonance, which delocalizes a radical over several atoms and reduces its instability enough to avoid undesired reactions (Griller & Ingold, 1976).
During the talk that took place on October 29th, Professor Nagib began by discussing his group’s work on the polarity reversal of functional group. Functional groups (such as ketones) are arrangements of atoms that have specific characteristics, regardless of the arrangement of other atoms in a molecule. In an electrically neutral form, ketones and other carbonyl (carbon-oxygen double bond)-bearing functional groups are naturally electrophilic, where electron rich groups such as alkenes attack and form new carbon-carbon bonds needed in the synthesis of complex molecules.
Professor Nagib noted that previous attempts to use nucleophilic, or electron rich ketyl radicals created from these carbonyl groups to perform carbon-carbon bonding required large excesses of electron-donating metals like sodium to overcome the ketyl’s tendency to reform the neutral ketone (caused by its very negative reduction potential of -2 volts) (Wang et al., 2018). However, he reported that his group had created a net neutral redox strategy for the production of ketyl radicals from aldehydes using the addition of acetyl iodide. The addition of an iodide ion from acetyl iodide to aldehydes, combined with the use of a manganese photocatalyst reduced the required redox potential by over 1 volt, made the chemical requirements for the formation of the ketyl radical significantly easier to satisfy. In experiments with larger substrates, this iodide and manganese-based method led to a 78% yield of desired vinyl iodides with a 20:1 selectivity favoring Z isomers over E isomers. While previous attempts at the synthesis of vinyl halides were successful for vinyl fluorides, chlorides, and bromides, Nagib’s lab presented the first efficient radical-based synthesis of the much more sterically hindered vinyl iodides (Koh et al., 2016; Nagib, 2020).
After discussing his work on polarity reversal with ketyl radicals, Nagib discussed his recent work on attempting to control site selective activation of carbon-hydrogen bonds–that is, the replacement of a C-H bond with a C-C, C-N, C-O, or carbon-halogen bond to introduce more reactive functional groups needed for the synthesis of complex molecules. He presented his group’s work on the C-H activation of arene and heteroarene rings with anions like chloride, bromide, mesylate, tosylate and triflate. Through the reaction with an asymmetric iodane, an organic derivative of the halogen iodine, Nagib demonstrated para-selective C-H activation for arenes and C3-selective activation of heteroarenes. He added that computational analysis suggested that this reaction proceeded through the formation of a radical cation intermediate in the arene ring. This reaction may play an important role in the synthesis of potential medicines, as according to Gal et. al the introduction of a halide ion or other anion can improve the medicine’s binding affinity through providing strong bonding interactions between a medicinal compound and a target molecule (Gál, Bucher, & Burns, 2016).
Finally, Nagib presented some of his most recent work on new synthetic methods for the production of piperidines and oxazoles (Nagib, 2020). He noted that all of these reactions are important for the development of new medicines; however, prior efforts to produce these molecules through radical intermediates did not have high yields or stereoselectivity. Starting with piperidine production, he said that through the use of a copper catalyst and Me3SiCN, his group was able to produce highly enantioselective activation of a delta C-H bond of an amine with a cyanide ion. Upon treatment with hydride ions, this produced the desired piperidines in 99% enantionmeric excess for the R isomer (Nagib, 2020). Nagib noted that the group achieved this result by trapping a one electron radical on the delta carbon with an acrylate ion to allow the formation of a carbon-carbon bond with the CN anion. With regard to the synthesis of oxazoles, he noted that traditional oxazole synthesis methods require the condensation of amides and ketones, but his group’s strategy made use of more commercially available alcohols and nitriles. First, the group produced imidates from the nitrile and alcohol starting products with the use of triflic acid. Then, through treatment of this intermediate with cesium iodide, PhI(OAc)2, and light, the group was able to produce the desired oxazoles with a 98% yield. This second step proceeded through a tandem hydrogen atom transfer, in which the beta carbon is first aminated before an intramolecular reaction occurs to close the ring, which is subsequently oxidized to the oxazole via a AcO radical (Nagib, 2020).
Nagib concluded his talk by mentioning that while the field of radical-based synthesis is still growing it shows great potential for the quick and efficient production of complex molecules. He added that because these radical based reactions avoid the need for the usage of toxic and rare metals like molybdenum or highly acidic conditions, these radical reactions might make organic synthesis more environmentally friendly.
References
Griller, D., & Ingold, K. U. (1976). Persistent carbon-centered radicals.Accounts of Chemical Research, 9(1), 13-19. doi:10.1021/ar50097a003
Gál, B., Bucher, C., & Burns, N. (2016). Chiral Alkyl Halides: Underexplored Motifs in Medicine.Marine Drugs, 14(11), 206. doi:10.3390/md14110206
Koh, M. J., Nguyen, T. T., Zhang, H., Schrock, R. R., & Hoveyda, A. H. (2016). Direct synthesis of Z-alkenyl halides through catalytic cross-metathesis.Nature, 531(7595), 459-465. doi:10.1038/nature17396
Nagib, D. (2020, November 3).C-H and C-O Functionalization via Radical Chaperones. Lecture.
Walling, C. (2020). Radical. Retrieved November 09, 2020, from https://www.britannica.com/science/radical-chemistry
Wang, L., Lear, J. M., Rafferty, S. M., Fosu, S. C., & Nagib, D. A. (2018). Ketyl radical reactivity via atom transfer catalysis.Science, 362(6411), 225-229. doi:10.1126/science.aau1777
Related Posts
Super Selective Synthesis: The Evolution of Enantioselective Methods
Figure 1: Two enantiomers of an amino acid. These molecules...
Read MoreFactors in the Onset of Obesity – An American Public Health Crisis
Figure 1: Obesity is typically diagnosed based on BMI. The...
Read MoreThe Chernobyl Accident — A Natural Experiment for the Effects of Radiation on Humans
Figure 1: A monument (front left) to the meltdown at...
Read MoreAndrew Sasser