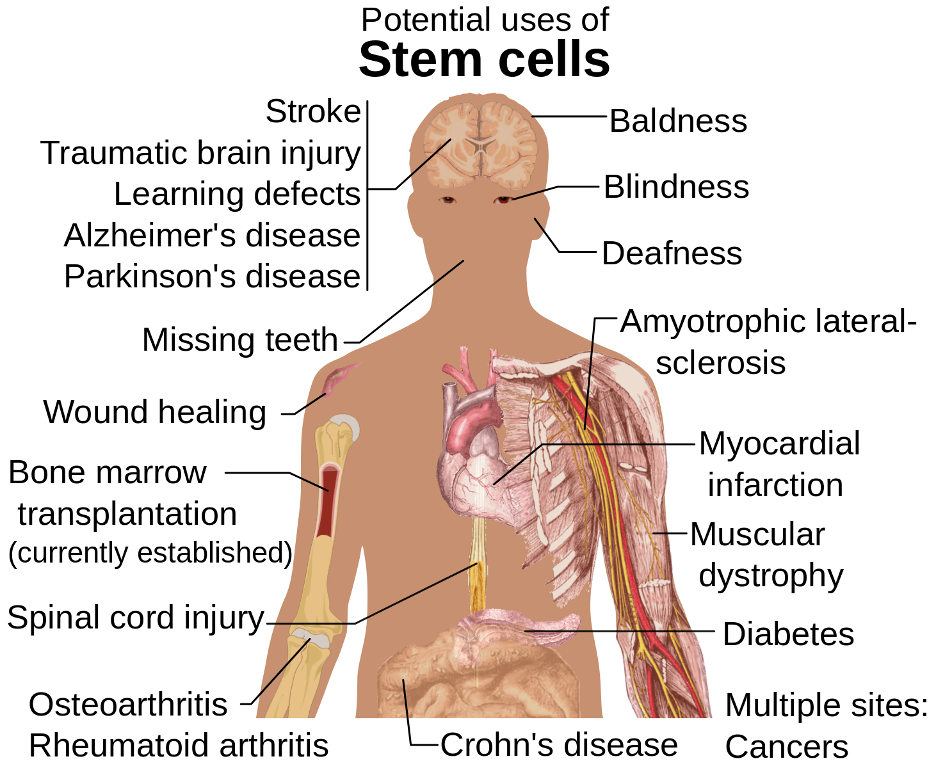
The diagram above displays the many potential uses of stem cells. To make the most use of stem cells’ versatility and life-saving capacity, researchers must develop a detailed understanding of where stem cells can be found and what roles they play in the body. (Source: Wikimedia Commons, Mikael Häggström)
The biology of stem cells, which can develop into any of a variety of different cell types in the body, is a popular area of study in the medical field. Researchers are already working to harness stem cells’ regenerative potential to enhance healing. For instance, Schmitt et al. (2015) developed a gel-based immobilization matrix system that can maintain stem cells at a localized surgical site to aid in recovery. Given the many potential uses of stem cells in therapeutic medicine, it is unsurprising that identifying the locations of stem cells in the body has been and continues to be a major initiative in the medical field. This is especially true of stem cells near the brain, which can differentiate into neurons, structurally supportive astrocytes, or glial cells, which help insulate the axons of nerve cells to allow for more rapid transmission of electrical signals in the nervous system. However, standard lab dissection methods like slicing bone tissue into lengthwise sections for visualization have proved ineffective when applied to the irregular, curved craniofacial bones that comprise the skull (Rindone et al., 2021).
In the past, scientists have gotten around the skull’s inconvenient geometry by mapping only small sections of blood vessels and stem cells in the brain, using the mouse skull as a model. However, a need remains for a more comprehensive map of the entire skull and the blood vessels and stem cells it contains (Johns Hopkins Medicine, 2021). Given that blood vessels and stem cells play critical roles in bone formation and healing, completing this map would be of immense value to neurosurgeons and other clinicians that deal with bone injuries.
A new technique developed by Rindone et al. (2021) makes the circulatory system within the skull more accessible to scientists. Rindone and colleagues have created a single-cell resolution, 3D map of the skull’s internal blood vessels and stem cells. So far, the team has mapped the top of the mouse skull, including four connected bones. To accomplish this, they tagged molecules that associate specifically with blood vessels and stem cells with fluorescent chemicals, relying on light-emitting molecules exclusively from the red to infrared spectrum to avoid the light scattering associated with ultraviolet and green dyes. The degree to which light scatters as it makes contact with air molecules depends on its wavelength. Generally, the shorter the wavelength, the more scattered the light will be. Therefore, selecting molecules that emit light with longer wavelengths (like red light) helped prevent the scattering that might have occurred with short-wavelength colors like green or ultraviolet. To achieve the tagging itself, Rindone et al. (2021) applied primary antibodies (blood proteins that target specific chemical substances called antigens) to the relevant tissue, using different antibodies to label CD31 receptors and Emcn proteins, which are expressed in and therefore mark general blood vessels, and Osterix and Gli1 proteins, which mark the skull stem cells. Secondary antibodies were then applied, with each binding to one of the previously used primary antibodies. Finally, the fluorescent markers were applied, and each paired with one of the secondary antibodies.
After introducing the dye-tagged compounds to color the blood vessels and stem cells, the researchers used an additional chemical compound that would further prevent light from scattering as it passed through the skull (Johns Hopkins Medicine, 2021). After taking the image with a light-sheet fluorescent microscope, which avoids photobleaching (a loss of fluorescence because of light-induced damage) by imaging in sections, Rindone et al. (2021) used a computer program to identify cellular structures and record relevant measurements like vessel volume, the number of stem cells present, and the distance between stem cell clusters and blood vessels. Blood vessel subtypes were differentiated by phenotype (observable traits resulting from gene expression) by the computer program using filtering algorithms.
This technique yielded several key insights. Excitingly, the 3D map revealed new stem cell locations in the skull, many of which are close to transcortical canals, channels in the skull bone bridging its outer lining and internal cavities containing bone marrow, where stem cells are already known to reside (Johns Hopkins Medicine, 2021). Additionally, Rindone et al. (2021) found that not all blood vessels are created equal, as bone cell precursors were most associated with the CD31hiEmcnhi blood vessel subtype. The research team found further evidence implicating this blood vessel subtype in bone development and healing when they found it to be associated with Gli1+ and Osterix+ cells, both of which have been previously established as key to bone growth. Importantly, this association was only observed in the stages of healing in which most bone growth occurs, and the regression of CD31hiEmcnhi blood vessels was associated with a healing stage in which little bone forms (Rindone et al., 2021).
Overall, Rindone et al. (2021) have made an essential breakthrough in imaging the skull and its contents, uncovering new stem cell populations and documenting relationships between blood vessel subtypes and precursors to bone cells in mice. They hope that their technique can be adapted to the study of the human skull in the near future and hypothesize that it can also be modified to investigate the unknown roles other cells like neurons and immune cells play in bone growth and healing (Rindone et al., 2021). If the technique is applied to human physiology, this could lead to a major advancement in surgical techniques. For instance, Glover et al. (2012) found that surgical corrections of skull disfigurements from traumatic brain injuries can do more harm than good in some cases. This is because abrupt aesthetic skull reconstructions can disrupt brain swelling that ordinarily relieves internal pressure, ultimately leading to larger cortical damage. In discovering new pockets of stem cells in the skull, Rindone et al. (2021) might have provided an avenue for doctors to induce natural bone growth by drawing from the newly identified stem cell populations for local application and stimulating CD31hiEmcnhi blood vessel proliferation rather than surgically patching up the skull in response to a traumatic brain injury. This alternative approach could provide the brain with sufficient time to swell and recover, limiting cortical damage. With research teams like Schmitt et al. (2015) already working to develop practical stem cell applications like the previously discussed immobilization matrix for surgical recoveries, the use of stem cells to treat localized skull damage might not lie too far off in the future. By opening the contents of the mouse skull for all to see, Rindone et al. (2021) may have also revealed a map for the future of stem-cell-based therapies.
Appendix
3D map of the top of the mouse skull as imaged by Rindone et al. (2021): https://www.youtube.com/watch?v=VGNdBcHNo7E
References
Glover, L. E., Tajiri, N., Lau, T., Kaneko, Y., Loveren, H. van, & Borlongan, C. V. (2012). Immediate, but not delayed, microsurgical skull reconstruction exacerbates brain damage in experimental traumatic brain injury model. PLoS One, 7(3), e33646. http://dx.doi.org/10.1371/journal.pone.0033646
Johns Hopkins Medicine. (2021, November 18). A stunning 3D map of blood vessels and cells in a mouse skull could help scientists make new bones. ScienceDaily. Retrieved December 7, 2021 from www.sciencedaily.com/releases/2021/11/211118203616.htm
Rindone, A. N., Liu, X., Farhat, S., Perdomo-Pantoja, A., Witham, T. F., Coutu, D. L., Wan, M., & Grayson, W. L. (2021). Quantitative 3D imaging of the cranial microvascular environment at single-cell resolution. Nature Communications, 12(1), 6219. https://doi.org/10.1038/s41467-021-26455-w
Schmitt, A., Rödel, P., Anamur, C., Seeliger, C., Imhoff, A. B., Herbst, E., Vogt, S., van Griensven, M., Winter, G., & Engert, J. (2015). Calcium alginate gels as stem cell matrix – Making paracrine stem cell activity available for enhanced healing after surgery. PLoS ONE, 10(3), e0118937. https://doi.org/10.1371/journal.pone.0118937
Related Posts
A New Hybrid Method for Sequencing the Melanoma Genome
Figure 1: Cell culture in a Petri dish Source: Wikimedia...
Read MoreStaphylococcus epidermidis capable of 6-HAP production reduce host UV-induced tumors
Figure 1: The human microbiome is the collection of microorganisms...
Read MoreDirecting Neural Stem Cells to Repair Damage
Figure 1: This depicts the ‘undifferentiated’ nature of pluripotent stem...
Read MoreMechanisms of Metabolic Robustness Discovered in E. Coli
Figure 1: This diagram demonstrated how CRISPR interference (CRISPRi) works...
Read MoreTrust: The Essence of Good Policy Implementation
Figure 1 President Tsai Ing-wen inspects the Central Epidemic Command...
Read MoreBrain Circuit Disorders in PTSD
Figure 1: PTSD and its comorbid mental health symptoms contribute...
Read MoreCaroline Conway