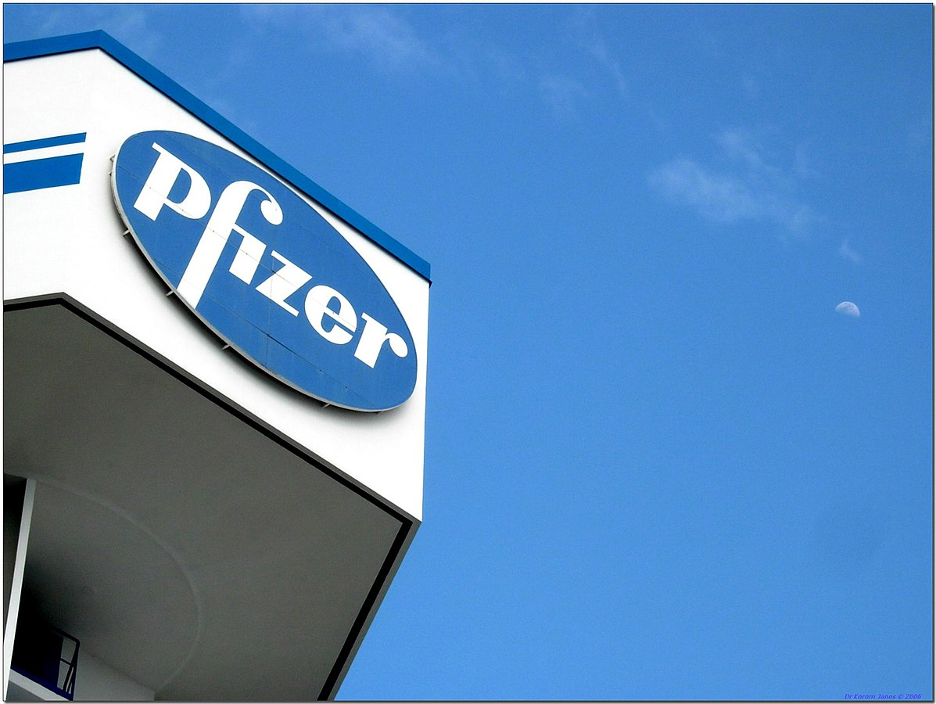
A photo of a Pfizer facility in Italy. (Image Source: Dr. János Korom, Wikimedia Commons)
On November 5th, 2021, Pfizer announced that it would seek FDA approval for its new drug, called Paxlovid. It is given to those with an active case of COVID-19 and has been shown to reduce the risk of hospitalization and death due to severe COVID-19 infections by 89% in a Phase 2/3 clinical trial (Pfizer, 2021). The drug consists of a combination of a SARS-CoV-2 (the virus that causes COVID-19) nsp5 inhibitor called PF-07321332 (see Figure 2, below) and an inhibitor of Cytochrome P450 3A4 (a human protein that often breaks down drugs) called Ritonavir, which has been used with other protease inhibitors in the past to prolong their half-life in vivo (Mahase, 2021; Owen et al., 2021).
Also known as Mpro or 3CLpro, nsp5 is a viral protease essential for coronavirus infection and replication in human cells. It is responsible for freeing eleven of the fifteen proteins encoded within the orf1ab polyprotein that a coronavirus produces by cutting at ten different cleavage sites. Many of these proteins go on to form the viral replicase complex that produces copies of the virus’s RNA genome (Fehr & Perlman, 2015; Perlman & Netland, 2009). Thus, in inhibiting nsp5, the polyprotein is not cleaved and the proteins that make up the viral replicase complex are not functional. So, when the protein is inhibited, the genome cannot be replicated, and no functional viral progeny can be produced. Essentially, if nsp5 is inhibited, once a virus infects a cell, no new viruses can be produced by that infected cell (Anand et al., 2003; Jin et al., 2020; Owen et al., 2021).
This is how Pfizer’s drug works: PF-07321332 binds to and inhibits nsp5, preventing the separation of the polyprotein and production of viable viral offspring while Ritonavir makes sure PF-073321332 isn’t degraded. Thus, in those who are infected with the virus, the internal viral load is reduced, and immune systems aren’t overwhelmed. Since an over-activated immune system has been linked to much of the organ damage seen in severe COVID-19 cases, keeping the immune system at an optimally activated level is key to clearing the virus without harming the host in the process (Yazdanpanah et al., 2020).
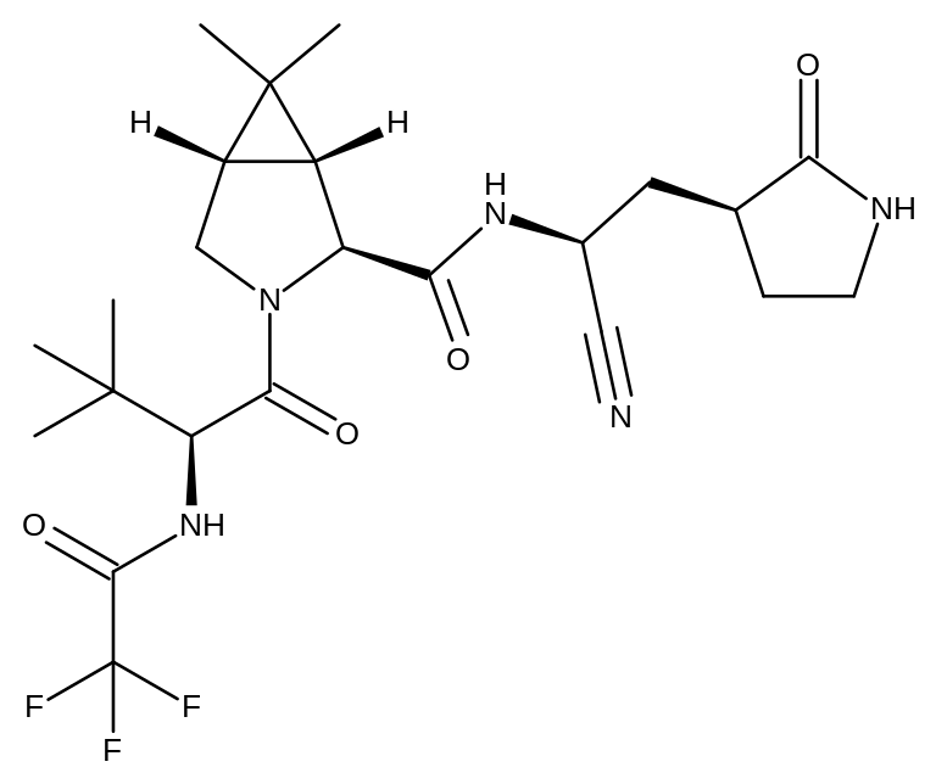
Figure 1: A carbon skeleton diagram of PF-07321332. (Image Source: Michael D. Turnbull, Wikimedia Commons)
Using PF-07321332’s to inhibit nsp5 is not a novel idea. The first drug of this type was an inhibitor of nsp5 from Feline Coronavirus (FCoV), a coronavirus that causes diarrhea and other intestinal problems in cats. Much like SARS-CoV-2 infections, FCoV infections often turn fatal, as the host’s immune system is hyperactivated and ends up causing widespread catastrophic damage (Pedersen, 2014). Due to the rate of fatality in infected cats, a drug called GC376 (see Figure 3, below) was developed with the purpose of treating FCoV infections by targeting nsp5. The chemical mechanism of the drug is as follows: GC376 is converted to a related compound within the body, called GC373, in which the bisulfite (SO2ONa) group (circled in Figure 3 below) is replaced by an aldehyde (COH) group. The catalytic cysteine residue of the nsp5 enzyme attacks the carbonyl (C=O) bond of this aldehyde group, resulting in the formation of a stable thiohemiacetal. In forming this enzyme-inhibitor complex, the enzyme active site is occupied, and the catalytic residue is modified, rendering nsp5 inactive (Kim et al., 2016).

Figure 2: A carbon skeleton diagram of GC-376. A circle has been added around the bisulfite group for emphasis. (Image Source: Meodipt, Wikimedia Commons)
Being safe and effective in cats, GC-376 and GC-373 were tested as potential inhibitors of nsp5 from SARS-CoV-2 (Pedersen et al., 2018). Not only were they seen to effectively bind to and inhibit the enzyme, but there also seemed to be no toxicity in human cell cultures (Vuong et al., 2020). Thus, the nsp5 enzyme structure must be largely conserved across the two coronaviruses. Notably, FCoV and SARS-CoV-2 are from different genera of the coronavirus family: alpha and beta, respectively (NCBI, 2005, 2020). It seems that the companies behind these two drugs came up with them separately despite the structural similarity between PF-07321332 and GC-373/376 (note the characteristic carbonyls and secondary amines, indicative of a peptide chain, and the γ-lactam on the far right of Figures 2 and 3: the 5 membered ring with a carbonyl and internal secondary amine). Thus, it is likely that PF-07321332/Paxlovid would also be an effective inhibitor of FCoV nsp5. This γ-lactam motif seems to mimic the amino acid glutamine (since it is a secondary amide). Notably, across the 10 nsp5 cleavage sites, the residue immediately before the cleavage site is always a glutamine. This glutamine is not only found within the orf1ab polyprotein of SARS-CoV-2, it is also found in the analogues of the orf1ab polyprotein in MERS-CoV, SARS-CoV, FCoV, and other coronaviruses) including members of alpha, beta, and gamma coronaviruses – delta coronaviruses are less researched so there are fewer to study) (Hegyi & Ziebuhr, 2002; Kiemer et al., 2004; Wu et al., 2015). Could the shared γ-lactam/glutamine-mimicking feature be leading to this cross-genera inhibition seen above? Further research into these drugs could expand on this idea and reveal how to optimize these drugs.
The four genera of coronaviruses (alpha, beta, gamma, and delta) differ in two ways. Firstly, they infect different hosts: alpha and beta infect only mammals, gamma and delta infect mostly birds and some mammals. Secondly, their genomic structures differ. These differences include the ordering of their various structural proteins and the types of accessory proteins (proteins related to immune evasion and pathogenesis) they contain. However, the nsp genes contained within the orf1ab polyprotein are left mostly unchanged (Cui et al., 2019). Thus, nsp5, along with its cleavage sites (especially that critical glutamine) are incredibly well-conserved. Therefore, not only can Paxlovid and other γ-lactam containing analogs serve as anti-coronaviral drugs for currently circulating coronaviruses, but it is also very likely that if and when the next pandemic-level coronavirus makes the zoonotic jump into humans, such a drug would be safe and effective against it as well.
With the recent news of the emergence of the troubling Omicron variant of SARS-CoV-2, many have been worried about whether our current vaccines and drugs (including Paxlovid) will be effective against it (Aleem & Kothadia, 2021; Callaway & Ledford, 2021; Roy, 2021). The fact that GC-373/376 is effective across coronavirus genera suggests that it and PF-07321332 will be effective against all variants of SARS-CoV-2; for example, one study found that while nearly half and of genomic changes in the Delta (47%) and Lambda (46%) variants were in the nsps, the Delta variant had no changes to nsp5 and none of the five lambda variant changes affected the catalytic cysteine or the active site (Moghaddar et al., 2021). Thus, while these viruses will inevitably change over time due to mutation and natural selection, these changes do not seem to be affecting the target sites of nsp5. And they are unlikely to even as the coronavirus mutates further, because any change would have to be reflected across all ten cleavage sites and within nsp5 itself; changing just one or even a couple would lead to an incorrectly spliced polyprotein and thus nonfunctional viral progeny. Since the likelihood of so many concerted changes occurring at once is quite low, the target sites of nsp5 have yet to be changed by evolution. At the time of writing this, no analysis of Omicron’s genome and the mutations within nsps has been done, but, given nsp5’s critical nature and the multiple mutations required, it is likely that any results would be similar to those found by Moghaddar et al.
The argument outlined above also indicates that it is very unlikely that these viruses will be able to develop resistance to these drugs. Despite the selection pressure such a drug may apply to the virus population, it is simply very unlikely that all of those changes will occur within one generation. This is especially true if the drug is given in a “cocktail” with other anti-coronaviral drugs (like the FDA approved Remdesivir or UK approved Moluniprivir (Willyard, 2021)); drug cocktails have long been used against diseases (like HIV and cancer) that are quick to develop drug resistance, because treating with multiple drugs means that a pathogen’s ability to adapt to one drug is inhibited by its need to adapt to the other(s) (Zimmer et al., 2016).
Given all of this, Pfizer’s Paxlovid has an incredibly promising future, not only to help treat victims of the current pandemic, but also of any future pandemics that may be caused by the inevitable zoonotic jumps of coronaviruses into human hosts (as has occurred with SARS, MERS, and now COVID-19).
References
Aleem, A., & Kothadia, J. (2021). Remdesivir. StatPearls. https://www.statpearls.com/ArticleLibrary/viewarticle/122861
Anand, K., Ziebuhr, J., Wadhwani, P., Mesters, J. R., & Hilgenfeld, R. (2003). Coronavirus Main Proteinase (3CLpro) Structure: Basis for Design of Anti-SARS Drugs. Science, 300(5626), 1763–1767. https://doi.org/10.1126/science.1085658
Callaway, E., & Ledford, H. (2021). How bad is Omicron? What scientists know so far. Nature. https://doi.org/10.1038/d41586-021-03614-z
Cui, J., Li, F., & Shi, Z.-L. (2019). Origin and evolution of pathogenic coronaviruses. Nature Reviews. Microbiology, 17(3), 181–192. https://doi.org/10.1038/s41579-018-0118-9
Fehr, A. R., & Perlman, S. (2015). Coronaviruses: An Overview of Their Replication and Pathogenesis. In H. J. Maier, E. Bickerton, & P. Britton (Eds.), Coronaviruses: Methods and Protocols (pp. 1–23). Springer. https://doi.org/10.1007/978-1-4939-2438-7_1
Hegyi, A., & Ziebuhr, J. (2002). Conservation of substrate specificities among coronavirus main proteases. Journal of General Virology, 83(3), 595–599. https://doi.org/10.1099/0022-1317-83-3-595
Jin, Z., Du, X., Xu, Y., Deng, Y., Liu, M., Zhao, Y., Zhang, B., Li, X., Zhang, L., Peng, C., Duan, Y., Yu, J., Wang, L., Yang, K., Liu, F., Jiang, R., Yang, X., You, T., Liu, X., … Yang, H. (2020). Structure of Mpro from SARS-CoV-2 and discovery of its inhibitors. Nature, 582(7811), 289–293. https://doi.org/10.1038/s41586-020-2223-y
Kiemer, L., Lund, O., Brunak, S., & Blom, N. (2004). Coronavirus 3CLpro proteinase cleavage sites: Possible relevance to SARS virus pathology. BMC Bioinformatics, 5, 72. https://doi.org/10.1186/1471-2105-5-72
Kim, Y., Liu, H., Galasiti Kankanamalage, A. C., Weerasekara, S., Hua, D. H., Groutas, W. C., Chang, K.-O., & Pedersen, N. C. (2016). Reversal of the Progression of Fatal Coronavirus Infection in Cats by a Broad-Spectrum Coronavirus Protease Inhibitor. PLoS Pathogens, 12(3), e1005531. https://doi.org/10.1371/journal.ppat.1005531
Mahase, E. (2021). Covid-19: Pfizer’s paxlovid is 89% effective in patients at risk of serious illness, company reports. BMJ, 375, n2713. https://doi.org/10.1136/bmj.n2713
Moghaddar, M., Radman, R., & Macreadie, I. (2021). Severity, Pathogenicity and Transmissibility of Delta and Lambda Variants of SARS-CoV-2, Toxicity of Spike Protein and Possibilities for Future Prevention of COVID-19. Microorganisms, 9(10), 2167. https://doi.org/10.3390/microorganisms9102167
NCBI. (2005). Feline infectious peritonitis virus. NCBI. https://www.ncbi.nlm.nih.gov/data-hub/taxonomy/11135/?utm_source=datasets&utm_medium=referral&utm_campaign=genome-table&utm_term=Feline%20infectious%20peritonitis%20virus
NCBI. (2020). Severe acute respiratory syndrome coronavirus 2 isolate Wuhan-Hu-1, complete genome (Version 2) [Data set]. http://www.ncbi.nlm.nih.gov/nuccore/NC_045512.2
Owen, D. R., Allerton, C. M. N., Anderson, A. S., Aschenbrenner, L., Avery, M., Berritt, S., Boras, B., Cardin, R. D., Carlo, A., Coffman, K. J., Dantonio, A., Di, L., Eng, H., Ferre, R., Gajiwala, K. S., Gibson, S. A., Greasley, S. E., Hurst, B. L., Kadar, E. P., … Zhu, Y. (2021). An oral SARS-CoV-2 Mpro inhibitor clinical candidate for the treatment of COVID-19. Science, 0(0), eabl4784. https://doi.org/10.1126/science.abl4784
Pedersen, N. C. (2014). An update on feline infectious peritonitis: Virology and immunopathogenesis. Veterinary Journal (London, England : 1997), 201(2), 123–132. https://doi.org/10.1016/j.tvjl.2014.04.017
Pedersen, N. C., Kim, Y., Liu, H., Galasiti Kankanamalage, A. C., Eckstrand, C., Groutas, W. C., Bannasch, M., Meadows, J. M., & Chang, K.-O. (2018). Efficacy of a 3C-like protease inhibitor in treating various forms of acquired feline infectious peritonitis. Journal of Feline Medicine and Surgery, 20(4), 378–392. https://doi.org/10.1177/1098612X17729626
Perlman, S., & Netland, J. (2009). Coronaviruses post-SARS: Update on replication and pathogenesis. Nature Reviews Microbiology, 7(6), 439–450. https://doi.org/10.1038/nrmicro2147
Pfizer. (2021, November 5). Pfizer’s Novel COVID-19 Oral Antiviral Treatment Candidate Reduced Risk of Hospitalization or Death by 89% in Interim Analysis of Phase 2/3 EPIC-HR Study. https://www.pfizer.com/news/press-release/press-release-detail/pfizers-novel-covid-19-oral-antiviral-treatment-candidate
Roy, M. (2021, November 30). Regeneron’s COVID-19 antibody drug may be less effective against Omicron. Reuters. https://www.reuters.com/business/healthcare-pharmaceuticals/regeneron-says-covid-19-drug-could-be-less-effective-against-omicron-variant-2021-11-30/
Vuong, W., Khan, M. B., Fischer, C., Arutyunova, E., Lamer, T., Shields, J., Saffran, H. A., McKay, R. T., van Belkum, M. J., Joyce, M. A., Young, H. S., Tyrrell, D. L., Vederas, J. C., & Lemieux, M. J. (2020). Feline coronavirus drug inhibits the main protease of SARS-CoV-2 and blocks virus replication. Nature Communications, 11, 4282. https://doi.org/10.1038/s41467-020-18096-2
Willyard, C. (2021). How antiviral pill molnupiravir shot ahead in the COVID drug hunt. Nature. https://doi.org/10.1038/d41586-021-02783-1
Wu, A., Wang, Y., Zeng, C., Huang, X., Xu, S., Su, C., Wang, M., Chen, Y., & Guo, D. (2015). Prediction and biochemical analysis of putative cleavage sites of the 3C-like protease of Middle East respiratory syndrome coronavirus. Virus Research, 208, 56–65. https://doi.org/10.1016/j.virusres.2015.05.018
Yazdanpanah, F., Hamblin, M. R., & Rezaei, N. (2020). The immune system and COVID-19: Friend or foe? Life Sciences, 256, 117900. https://doi.org/10.1016/j.lfs.2020.117900
Zimmer, A., Katzir, I., Dekel, E., Mayo, A. E., & Alon, U. (2016). Prediction of multidimensional drug dose responses based on measurements of drug pairs. Proceedings of the National Academy of Sciences, 113(37), 10442–10447. https://doi.org/10.1073/pnas.1606301113
Related Posts
The Geography of Disease: a Bayesian Approach to Epidemiology
Figure 1: A map showing relative rates of pancreatic cancer...
Read MoreAutism Spectrum Disorder and Gender: The Case for Expanding the Autistic Phenotype
This article was originally submitted to the Modern MD competition...
Read MoreBeyond the White Coat Worker: An Overview of Citizen Science
By: Olivia Brado1, Luz Mariana Cumpa Gomez1, Shirin Dadina1, Katrina...
Read MoreAn International Team of Researchers Just Solved One of the Biggest Mysteries About the Human Heart
Figure 1: The heart is one of the most complex...
Read MoreExploring Possibilities for the Early Diagnosis of Alzheimer’s Disease
Figure 1: Extracellular deposits of amyloid-beta proteins as seen in...
Read MoreThe Chernobyl Accident — A Natural Experiment for the Effects of Radiation on Humans
Figure 1: A monument (front left) to the meltdown at...
Read MoreFrankie Carr