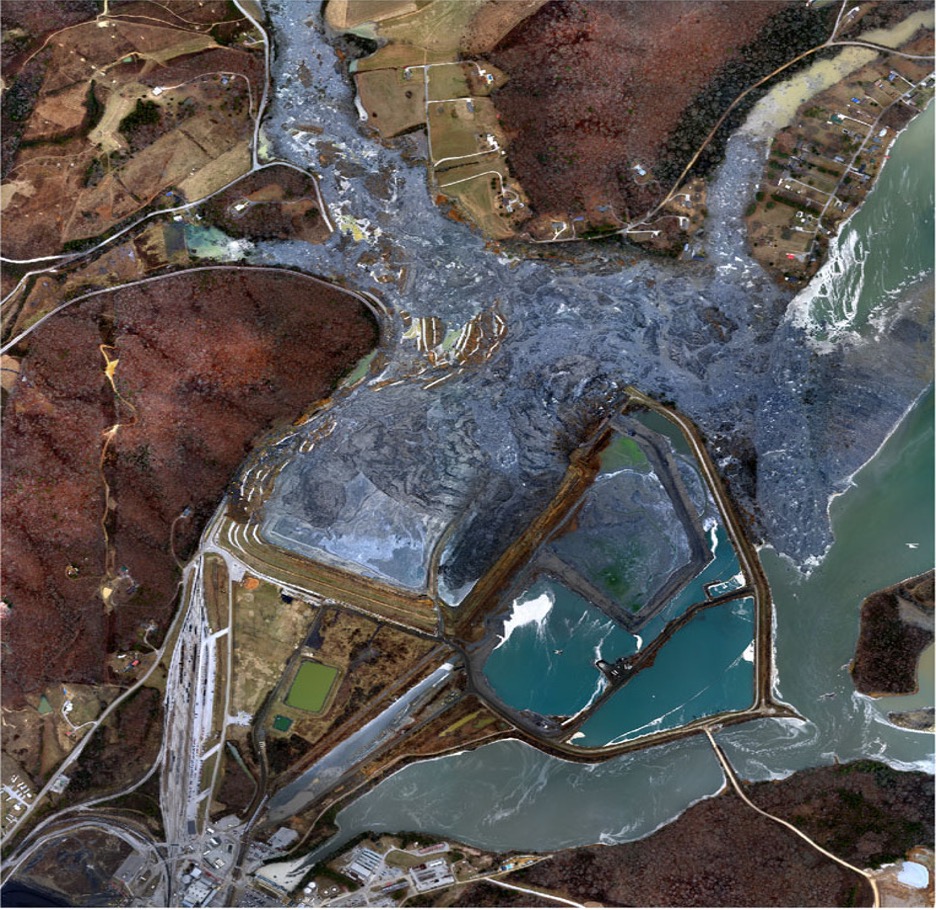
Figure 1: Aerial view of the 1.1 billion gallons of fly ash slurry spilled outside the Kingston Fossil Plant in Kingston, Tennessee. With geopolymer cement rising in popularity, spills like this will be preventable as fly ash is a promising geopolymer precursor, taking them out of quarries and into industry.
Source: Wikimedia Commons
When you think of climate change, cement is rarely the first culprit to come to mind; yet, the cement industry is responsible for 8% of anthropogenic CO2 emissions: an undeniable carbon footprint (Andrew, 2019). In 2018 alone, the cement industry’s global emissions amounted to approximately 1.50 Gt CO2, putting the production of Ordinary Portland cement (OPC) just behind fossil fuels in greenhouse gas emissions (Andrew, 2019; Behzad & Sanjayan 2014). These high emission rates are caused by the combustion reactions that heat cement kilns, as well as the calcination of limestone inside the kilns. Considering classical cementitious mixtures such as concrete, mortar, stucco, and grout rely on OPC paste as a binder, these emission rates are unlikely to diminish anytime soon. Rather than attempting to redesign the cement industry’s entire energy consumption method, soft matter physicists and materials engineers have developed an alternative cementitious paste that eliminates the need for OPC altogether: geopolymers.
Taking notice of the abundance of aluminosilicate-rich industrial byproducts in the world, researchers have demonstrated a way to upcycle these wastes into alkali-activated cementitious pastes, also known as geopolymers, with similar or better mechanical properties than OPC pastes. Historically, byproducts from coal burning, ore smelting, or refining have been discarded into quarries and freshwater ecosystems which, in the case of Hungary’s Marcal river, has extinguished all proximal life (Mayes et al., 2016). Efforts to contain these byproducts will sometimes lead to spillage as in the 2008 Kingston Fossil Plant Disaster, pictured in Figure 1, which released 1.1 billion gallons of fly ash into the surrounding community. Using these waste materials as precursors to geopolymer binders prevents their discard into the environment, and renders OPC obsolete, effectively resolving cement-related ecosystem invasion and greenhouse gas emissions.
Much like classical binders, geopolymer binders are a mixture of solid and liquid substances that gelate and harden. Instead of OPC, the solids in geopolymer binders are alkali activated precursors such as fly ash, ground-granulated blast-furnace slag (GGBFS), bauxite residue (red mud), or other aluminosilicate-rich waste products. In place of water, geopolymers use activating solutions, typically alkali hydroxide solutions such as NaOH, KOH or alkali silicate solutions such as waterglass. Much like water hydrates Portland cement into a solid, activating solution reacts with geopolymer precursors to harden the paste. An advantage to these geopolymers is that some mixtures have excellent compressive strengths and are often more resistant to structural changes from chemical exposure than OPC binders (Bakharev, 2005; Wang et al., 2016). Though a promising solution, geopolymers are still in a developmental stage and thus are not yet commercially popular.
Major concerns holding back the implementation of geopolymers are the practicality of alkali activating solutions and the difficulty in maximizing their compressive strengths without compromising their viscosity. The practicality of activating solutions is a serious consideration since they are more expensive and volatile than water, the only liquid required to harden an OPC paste. If there was a way to reduce the amount of activating solution relative to the precursor (l/s ratio) in a geopolymer mixture, we could reduce the price and risk of this alternative cement; moreover, we would increase the compressive strength of the paste, accounting for both concerns (Han et al., 2011; Patankar et al., 2013). The problem with minimizing the l/s ratio is that, as the compressive strength increases, the fluidity, and thus the workability, decreases (Han et al., 2011; Patankar et al., 2013).
The typical solution to this fluidity-compressive strength trade-off in classical systems is the addition of superplasticizer. This additive frees liquid trapped in cement floccules through electrostatic and steric repulsion between the engulfing cement particles. Unfortunately, superplasticizers break down in very alkaline media (pH ~13) such as geopolymers, and are therefore ineffective additives (Palacios & Puertas, 2004). Though there are some geopolymers, such as fly ash C and red mud, in which polycarboxylate-based superplasticizers are proven to be more effective than others, it is not comparable to the effect seen in OPC (Alrefaei et al., 2019; Xie & Kayali, 2016).
The most promising solution to superplasticizer fragility in very alkaline media seems to be found in metakaolin geopolymers. It has been shown that the addition of sand as an inert filler in metakaolin geopolymer paste increases its cured compressive strength (Kuenzel et al., 2014). On the other hand, increasing the purity of the metakaolin precursors has proven to maximize fluidity with a given l/s ratio (Autef et al., 2013). From a thermodynamic approach, decreasing the setting temperature of metakaolin activated by NaOH has also been shown to increase fluidity (Romagnoli et al., 2012). These three techniques show promise in improving geopolymer quality without the need for superplasticizer. Though promising, they are specific to metakaolin which is only one of many geopolymers; thus, scientists are still looking for a universal solution.
State of the art research is being conducted at Katholieke Universiteit Leuven, Belgium, where materials engineers are manipulating surface properties of red mud precursors in order to change their surface energy. Whether through chemical etching, UV light exposure, or Corona treatment, the precursor’s surface energy will increase, in theory creating a superplasticizing effect without adding a superplasticizer itself. A possible challenge to this technique is cracking due to shrinkage from an increase in negative Laplace pressure, forcing capillary drainage in the cement. This problem will only occur in precursors which are hydrophobic prior to surface treatment. If a precursor is hydrophilic prior to surface microstructuring, there are two states which the surface can enter—Wenzel and Cassie-Baxter—each leading to different contact angles, and thus two different changes in Laplace pressure. The only, and most likely, case which is theorized to prevent shrinkage cracking is the Cassie-Baxter state, where the liquid-precursor interface is restrained to the topmost surface of deformation allowing for an increase in contact angle.
Ultimately, geopolymers are a promising new cement that will eliminate cement-industry-related emissions as well as minimize ecosystem pollution. Though work remains to be done before geopolymers can become commercially available, they are becoming much more popular. Finding ways to reduce l/s without affecting fluidity is the next step for this field, and future solutions will lead to great progress in valorizing geopolymer cement pastes.
References
Alrefaei, Y., Wang, Y.-S., & Dai, J.-G. (2019). The effectiveness of different superplasticizers in ambient cured one-part alkali activated pastes. Cement and Concrete Composites, 97, 166-174.
Andrew, R. M. (2019). Global CO2 emissions from cement production, 1928–2018. Earth System Science Data, 11(4).
Autef, A., Joussein, E., Poulesquen, A., Gasgnier, G., Pronier, S., Sobrados, I., . . . Rossignol, S. (2013). Influence of metakaolin purities on potassium geopolymer formulation: the existence of several networks. Journal of colloid and interface science, 408, 43-53.
Bakharev, T. (2005). Resistance of geopolymer materials to acid attack. Cement and concrete research, 35(4), 658-670.
Behzad, N., Sanjayan, J. (2014). Efficacy of Available Superplasticizers on Geopolymers. Research Journal of Applied Sciences, Engineering and Technology, 7.
Han, S., Yan, P., & Kong, X. (2011). Study on the compatibility of cement-superplasticizer system based on the amount of free solution. Science China Technological Sciences, 54(1), 183-189.
Kuenzel, C., Li, L., Vandeperre, L., Boccaccini, A., & Cheeseman, C. (2014). Influence of sand on the mechanical properties of metakaolin geopolymers. Construction and Building Materials, 66, 442-446.
Palacios, M., Puertas F. (2004). Stability of superplasticizer and shrinkage-reducing admixtures Stability of superplasticizer and shrinkage-reducing admixtures in high basic media. Materiales de Construcción, 54(274).
Mayes, W. M., Burke, I., Gomes, H. I., Anton, Á., Molnár, M., Feigl, V., & Ujaczki, É. (2016). Advances in understanding environmental risks of red mud after the Ajka spill, Hungary. Journal of sustainable metallurgy, 2(4), 332-343.
Patankar, S. V., Jamkar, S. S., & Ghugal, Y. M. (2013). Effect of water-to-geopolymer binder ratio on the production of fly ash based geopolymer concrete. Int. J. Adv. Technol. Civ. Eng, 2(1), 79-83.
Romagnoli, M., Leonelli, C., Kamse, E., & Gualtieri, M. L. (2012). Rheology of geopolymer by DOE approach. Construction and Building Materials, 36, 251-258.
Wang, W.-C., Wang, H.-Y., & Tsai, H.-C. (2016). Study on engineering properties of alkali activated ladle furnace slag geopolymer. Construction and Building Materials, 123, 800-805.
Xie, J., Kayali, O. (2016). Effect of superplasticiser on workability enhancement of Class F and Class C fly ash-based geopolymers. Construction and Building Materials, 122, 36-42.
Related Posts
Modeling Maximum Running Speed in Animals
Figure: Two cheetahs lie side by side. As the fastest...
Read MoreInsect Microbiome Gives Hope for New Antibiotic Discovery
Figure 1: Actinobacteria were isolated from 56% of the insect...
Read MoreThere’s NO Telling What Nitric Oxide Might Bring to Alzheimer’s Disease Research
Figure 1: As Alzheimer’s disease research begins to shift away...
Read MoreNicholas Patino