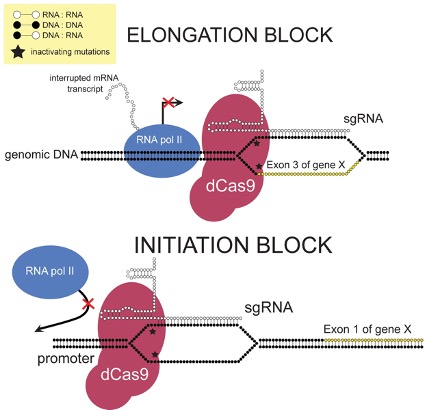
Figure 1: This diagram demonstrated how CRISPR interference (CRISPRi) works to inhibit the expression of certain genes at the transcription level. This mechanism has been used to study how changes in the expression of certain enzymes impacts a cell’s metabolism.
Source: Wikimedia Commons
The strength of enzymes present in a bacterium plays a significant role in its ability to grow and maintain homeostasis – a “steady state” that allows for optimal cell function. However, many bacteria like E. Coli are able to adapt their metabolic network (all enzyme-catalyzed reactions executed by a cell) to overcome either deficits in external nutrients or defects in the genome that inhibit the production of certain enzymes (Max-Planck-Gesellschaft, 2020). Previous studies had demonstrated that “knocking out,” or completely deleting, a single gene encoding an enzyme could lead to drastic changes in the concentrations of the molecules, more than 7000 in total, required for E. Coli metabolism (Fuhrer et al., 2017). However, as these gene deletions are permanent, these studies did not reveal how more moderate and dynamic changes, such as changes in nutrient sources, affect metabolism. Some scientists have suggested that these moderate changes should not drastically change the entire metabolic network, thereby enabling metabolic robustness – the ability for a metabolic network to react to enzymatic changes without significant impact to its functions. Until recently, no studies had directly measured metabolic robustness against changes in enzyme activity (Donati et al., 2020).
However, recent work by Donati et al. revealed how E. Coli is able to circumvent limitations to some of its metabolic pathways. The group used a method called CRISPR interference (CRISPRi) to enable sequence-specific control of gene expression (Donati et al., 2020). CRISPRi is derived from CRISPR-Cas9, a sort bacterial immune system that helps detect and fight off invading bacteriophages (viruses that infect bacteria). It has become popular in recent years as a successful gene editing tool. When expressed with a guide RNA, a protein from the CRISPR pathway can recognize certain DNA segments. Standard CRIPSR-Cas9 works to edit the DNA sequence at this point. CRISPRi works by interfering with the regular transcription of DNA to the mRNA needed to build proteins (Qi et al., 2013).
Using this technique, Donati et al. were able to generate some 7177 different strains of E. Coli, with random genes suppressed in each strain. Interestingly, the group found that most gene suppressions had little overall effect on the metabolism of E. Coli. There was some effect, though, on genes specifically related to metabolism. The suppression of seven such genes, such as the nrdAB operon needed for the synthesis of deoxyribonucleotide triphosphates (required for DNA replication), led to significant slowdowns in the rate of protein synthesis after just 4 hours. However, E. Coli cultures that had any of the other 1506 metabolism-related genes suppressed did not experience slowdowns in protein synthesis until almost 8 hours after the CRISPRi was induced (Donati et al., 2020).
Next, Donati et. al decided to investigate the mechanism through which E. Coli was able to circumvent the CRISPRi gene suppressions by comparing differences in the proteome (the sum of all proteins and enzymes present), as well as the metabolome (all metabolic molecules present in a strain) to that of an unimpacted E. Coli strain. Through this comparison, the group discovered that the suppression of certain enzymes led to the upregulation of other enzymes. For example, in response to the suppression of homocysteine transmethylase needed for the synthesis of methionine, the group detected higher than normal levels of other enzymes in the methionine synthesis pathway. The group also noted that some suppressed enzymes were re-activated by other molecules; for example, abnormally high levels of the amino acid ornithine were able to activate the suppressed carAB enzyme by binding to it (Donati et al., 2020). While the group only investigated 30 of the CRISPRi strains for changes in metabolic pathways, co-author Hannes Link noted that the group is working on developing new mass spectrometry techniques to determine quantitative changes for metabolites in all CRISPRi strains. Link also said that further understanding of metabolic robustness, which is necessary for the practice of metabolic engineering, could allow for improvements in the industrial biosynthesis of desirable molecules (Max-Planck- Gesellschaft, 2020).
References
Donati, S., Kuntz, M., Pahl, V., Farke, N., Beuter, D., Glatter, T., . . . Link, H. (2020). Multi-omics Analysis of CRISPRi-Knockdowns Identifies Mechanisms that Buffer Decreases of Enzymes in E. coli Metabolism.Cell Systems. doi:10.1016/j.cels.2020.10.011
Fuhrer, T., Zampieri, M., Sévin, D. C., Sauer, U., & Zamboni, N. (2017). Genomewide landscape of gene–metabolome associations in Escherichia coli.Molecular Systems Biology, 13(1), 907. doi:10.15252/msb.20167150
Max-Planck-Gesellschaft. (2020, November 24). Tackling metabolic complexity. Retrieved November 30, 2020, from https://www.mpg.de/16056749/1124-terr-x-tackling metabolic-complexity-153410
Qi, L., Larson, M., Gilbert, L., Doudna, J., Weissman, J., Arkin, A., & Lim, W. (2013). Repurposing CRISPR as an RNA-Guided Platform for Sequence-Specific Control of Gene Expression.Cell, 152(5), 1173-1183. doi:10.1016/j.cell.2013.02.022
Related Posts
Bispecific Antibody Recruits Vγ9+ γδ T cells for Leukemia Treatment
Figure 1: The image is taken from an elderly woman...
Read MoreProteins that Create “Speckles” in Cell Nuclei Discovered
Figure 1: A fluorescent microscopic image of the localization of...
Read MoreGut Bacteria May be a Cause of Colorectal Cancer (and the Key to Effective Treatment)
Figure: Cancer cell division is rapid and uncontrollable. New evidence...
Read MoreAndrew Sasser
Comments are closed.