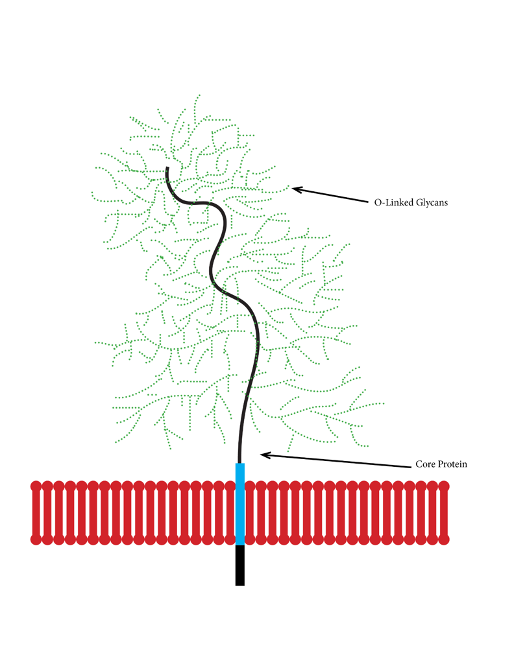
Figure: Diagram of Mucin Structure. Mucins are glycosylated membrane proteins with complex o-glycans on their extracellular TR region, which provide identifying information for interactions with microbes (Source: Jcastr07, Wikimedia Commons).
While the microbiome has been receiving increasing attention as a key physiological player, the mucinome, or mucus composition in the body, may be the gateway for researchers aiming to influence microbiome health (Wilkinson et al., 2021; Nason et al., 2021). In a recent study, scientists at the DNRF Centre of Excellence, Copenhagen Center for Glycomics, have developed a new method of reproducing the information in the mucinome. Through their efforts, they aim to provide the potential to therapeutically modify the microbiome, thus offering a medical intervention for various health conditions (Nason et al., 2021).
Mucus, or mucins, are glycosylated proteins (modified by carbohydrates as a form of identification) that form the sticky film interface between the digestive tract and the gut microbiome (Nason et al., 2021). They are also found in the lungs (which has its own unique microbial community), and in orifices, such as the eyes, which are prone to bacterial exposure (University of Copenhagen, 2021). The primary role of mucins is to act as a microbial “filter”– certain bacteria can bind to mucins through adhesive interactions and degrade mucin O-glycans to use as a nutrient supply (Nason et al., 2021). On the other hand, mucins are known to clear unwanted bacteria by disrupting bacterial biofilms (whole communities of bacterial cells that aggregate together), although the mechanism is still poorly understood. Because of the highly selective powers of the mucinome, it may be a powerful tool in therapeutically modifying the microbiome to boost human health (Nason et al., 2021).
With that selectivity, however, comes great complexity and specificity. Mucins are a highly heterogeneous group of macromolecules, varying greatly even at the species level (Nason et al., 2021). Furthermore, each mucin molecule is large and occupied by dense O-glycan motifs which can be difficult to accurately replicate using synthetic technologies. This poses a significant obstacle to mucinomics: prevailing methods of mucin reproduction such as recombinant expression in cell lines are limited by the detail and accuracy of the engineered plasmids used. Specifically, there are 18 mucin-associated genes for membrane or secreted mucins. Due to such obstacles, mucin study has been largely confined to isolated and synthetic O-glycans and short glycopeptides rather than full mucin structures, preventing scientists from studying mucins in their full context (Nason et al., 2021).
Nason and colleagues explain that using nascent facile nuclease-based gene engineering technologies, scientists can manufacture more complex and accurate plasmids instructing the cellular expression of mucin-associated glycogens (2021). Additionally, the ability to engineer both knock-out and knock-in variants of glycosylation genes within one plasmid allows scientists to explore glycan-dependent biological interactions. Because these experiments are done in an in vitro setting with cultured cells, there is the advantage of expressing glycans in their natural context of glycoproteins on the cell surface (Nason et al., 2021).
In Nason and colleagues’ experiments, the feature of focus is the tandem-repeat (TR) regions of the mucin glycans (2021). The TR regions are extracellular segments of the mucins featuring repeating patterns of O-glycans with great diversity of structure and position. These “glycocodes” comprise what is known as the mucinome, the informational hub for communication with microbes (Nason et al., 2021). Notably, compared with typical human extracellular oligosaccharides, TR regions are complex and variable enough to provide a greater binding epitome for the microbiota (Nason et al., 2021).
Using human embryonic kidney (HEK293) cells, the researchers were able to develop a library of the cell membrane and secreted mucin TR reporters (Nason et al., 2021). Defined TR regions programmed into the cells were tagged using green fluorescent protein (GFP). Notably, the expressed mucins were identifiable using mass spectroscopy, subverting the obstacles of alternative analytical methods. Additionally, the mucins were highly homogenous, leading scientists to speculate they could one day be mass-produced much like antibodies are today (Nason et al., 2021; University of Copenhagen, 2021).
Applications of great interest include therapeutically harnessing these lab-produced mucins for the enhancement of oral medication uptake, which is limited by the ability for the medication to attach to and be absorbed into the intestinal wall (University of Copenhagen, 2021). It is thought that if scientists can mimic bacterial adhesion to mucins in medications, they may bind more readily to the digestive wall and be more effective (University of Copenhagen, 2021).
Additional applications of Nason and colleagues’ work include the potential ability to modify the gut microbiome using specifically-tailored mucins as prebiotic material, encouraging the growth of beneficial bacteria in the gut (University of Copenhagen, 2021). This one application has potential uses across several medical fields, where conditions from neurodegeneration to cancer are being investigated in relation to the microbiome and pro- and prebiotic therapies are emerging as a promising form of alternative therapy (Peterson, 2020; Ge et al., 2021; Slizewska et al., 2021).
Mucins may also prove useful as an alternative to antibiotic use, given their ability to flush out harmful bacteria (University of Copenhagen, 2021). For example, one of the authors of the study proposes that lab-produced mucins may be administered as eye drops to help clear eye infections (University of Copenhagen, 2021). Given the ongoing battle against antibiotic-resistant bacteria, this application could be of particular importance (Andersson, 2003).
Finally, the authors note that viruses, in addition to bacteria, adhere to mucins (University of Copenhagen, 2021). When a virus proliferates, it tries to outcompete the mucins working to flush it out. Knowing that the influenza virus binds to a sugar found in mucins, scientists have already developed a sugar treatment, and there is hope that a mucin treatment may be even more effective (University of Copenhagen, 2021).
With groundbreaking applications in medical hot-topics such as medication absorption, the microbiome, and antibacterial and antiviral activities, the mucinome could be the next big thing in medical research (University of Copenhagen, 2021). Nason and colleagues’ groundbreaking work paves the way for scientists to study mucins as a powerful therapeutic of the future, and it will be exciting to watch as their findings serve as the foundation for many innovative technologies to come.
References
- Andersson, D. I. (2003). Persistence of antibiotic resistant bacteria. Current Opinion in Microbiology, 6(5), 452–456. https://doi.org/10.1016/j.mib.2003.09.001
- Ge, Y., Wang, X., Guo, Y., Yan, J., Abuduwaili, A., Aximujiang, K., Yan, J., & Wu, M. (2021). Gut microbiota influence tumor development and Alter interactions with the human immune system. Journal of Experimental & Clinical Cancer Research, 40(1), 42. https://doi.org/10.1186/s13046-021-01845-6
- Mucus and mucins may become the medicine of the future. (n.d.). ScienceDaily. Retrieved July 20, 2021, from https://www.sciencedaily.com/releases/2021/07/210707112413.htm
- Nason, R., Büll, C., Konstantinidi, A., Sun, L., Ye, Z., Halim, A., Du, W., Sørensen, D. M., Durbesson, F., Furukawa, S., Mandel, U., Joshi, H. J., Dworkin, L. A., Hansen, L., David, L., Iverson, T. M., Bensing, B. A., Sullam, P. M., Varki, A., … Narimatsu, Y. (2021). Display of the human mucinome with defined O-glycans by gene engineered cells. Nature Communications, 12(1), 4070. https://doi.org/10.1038/s41467-021-24366-4
- Peterson, C. T. (2020). Dysfunction of the Microbiota-Gut-Brain Axis in Neurodegenerative Disease: The Promise of Therapeutic Modulation With Prebiotics, Medicinal Herbs, Probiotics, and Synbiotics. Journal of Evidence-Based Integrative Medicine, 25, 2515690X20957225. https://doi.org/10.1177/2515690X20957225
- Śliżewska, K., Markowiak-Kopeć, P., & Śliżewska, W. (2020). The Role of Probiotics in Cancer Prevention. Cancers, 13(1), 20. https://doi.org/10.3390/cancers13010020
- Wilkinson, J. E., Franzosa, E. A., Everett, C., Li, C., Hu, F. B., Wirth, D. F., Song, M., Chan, A. T., Rimm, E., Garrett, W. S., & Huttenhower, C. (2021). A framework for microbiome science in public health. Nature Medicine, 27(5), 766–774. https://doi.org/10.1038/s41591-021-01258-0
Related Posts
Advances in the Search for an Alzheimer’s cure
Cover Image: This image displays the differences between a normal...
Read MoreUniversality of the Face: New Research Points to a Shared Human Commonality in Emotional Expression
Figure 1: Examples of emotional expressions Source: Andrea Piacquadio from Pexels Raising...
Read MoreThe Future of Wearable Devices
Figure: The colored sections of the brain represent the different...
Read MoreBad dreams? According to new studies, COVID-19 could be to blame
Figure 1: A sleeping child. When we sleep, we dream,...
Read MoreLearned Control of Spontaneous Dopamine Impulses in Mice
Figure: Dopamine is the so-called “feel-good” neurotransmitter, involved in cognitive...
Read MoreJillian Troth