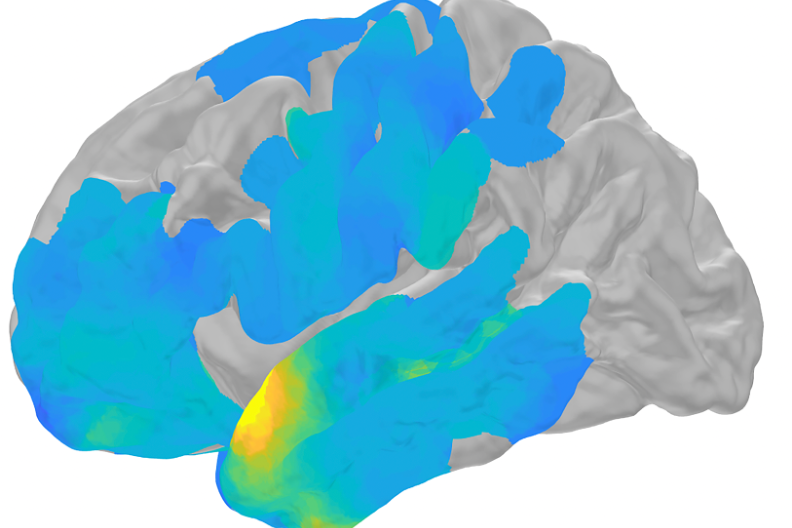
Figure: The colored sections of the brain represent the different areas that are activated by different stimuli (Source: Wikimedia Commons, NIH Image Gallery)
The field of computational neuroscience has made several advances in the last twenty years, including the design of a multitude of new technologies that stand to improve the quality of human life. The most prevalent commercially available wearable devices are smart watches which, at their most sophisticated, can monitor blood oxygen levels and sugar levels (HIMSS, 2021). In another class of wearables are Google’s Smart Lenses, which have sensors to detect internal changes, especially for individuals who are prone to developing diseases such as diabetes (Marr, 2021). For example, this device uses the tears from users’ eyes to process their blood sugar levels and identify if any action needs to be taken. All of these devices focus on data collection and analysis, but a new creation which could truly alter the lives of disabled individuals is a novel wearable brain-machine interface (BMI) system (Georgia Institute of Technology, 2021). A BMI system translates information from an individual’s neuronal activity and converts it to a command for a computer-operated or robotic limb. This new technology can greatly improve the lives of individuals who are paralyzed or struggle from motor dysfunction issues. The device could even allow people with locked-in syndrome to move based on an intention they have in their minds. More than data analysis and acquisition, this creation can cause an individual to physically act.
BMI devices have been built in the past, but they wouldn’t be classified as wearables – often requiring surgical implantation and a wired connection with an external computer. But now, a team of scientists led by Woon-Hong Yeo at the Georgia Institute of Technology have created a device with wireless soft scalp electronics and a powerful algorithm that enables their intentions to be turned into a physical action (Georgia Institute of Technology, 2021). Comparatively, Elon Musk’s company, Neuralink, claims to be making a surgically implantable chip into disabled individual’s brains in order to enable physical movement (Studio, 2016). Musk claims this technology is superior to other devices being developed, but the advantage to having a wearable device is its removability. The surgical implantation chip is more permanent and prone to hacks, which could damage the health of the user even further. In light of these risks, the wearable being developed by Yeo’s team is much safer for the individuals who require its use. The method in which these brain signals are collected is through electroencephalography (EEG). This is a diagnostic testing tool to measure and record the brain’s electrical activity using electrodes that are placed on an individual’s scalp. It’s a non-invasive method to examine the possibility of brain trauma or damage.
And the BMI system also has a virtual reality component – a set of wearable goggles – that allows the user to see a visual space where one, specific physical action is occurring, which prompts the subject to replicate the action and allows the researchers to gather accurate data. The surrounding virtual reality is not emphasized, it is often a singular color in order to draw attention to the physical action occurring in the virtual demonstration. The data collected records the motor imagery activity of the subject’s brain (Georgia Institute of Technology, 2021).
This new system has only been tested with four human subjects thus far, but the scientists who are working on the project hope to test it with disabled individuals in the future. While this technology is still being tested in laboratory and clinical settings at this time, it shows immense potential for the field of computational neuroscience and could dramatically improve the lives of disabled individuals (Georgia Institute of Technology, 2021).
In this study, the researchers monitored extrasynaptic dopamine levels in the primary somatosensory cortex (where both deterministic and spontaneous dopaminergic signaling occur) using fluorescence resonant energy transfer (FRET), a type of fluorescent imaging used to show the localization of a tagged molecule (Foo et al., 2021; Sekar & Periasamy, 2003). Specifically, implanted cell-based neurotransmitter fluorescent-engineered reporters (CNiFERs) were used to produce an optical signal observed using in vivo two-photon microscopy (Foo et al., 2021).
The researchers first distinguished spontaneous dopamine impulses in the absence of a reward, observing a wide distribution of both impulse length and amplitude (Foo et al., 2021). Then, they measured spontaneous dopamine impulses at baseline (no reward), given feedback (sucrose-water given in response to increased [DA]ex), then without feedback again (no reward). Within the second stage, referred to as the “feedback ON” stage, the researchers observed changes in [DA]ex both when the mice were rewarded simply for [DA]ex above a determined baseline and when the reward threshold either increased or decreased in accordance with [DA]ex change (Foo et al., 2021).
By the second day of feedback training, the researchers observed a statistically significant increase in [DA]ex (Foo et al., 2021). When the feedback was removed on day 4, the [DA]ex level returned to baseline, but it was restored with a return of feedback on day 5, demonstrating that the mice’s learned ability to volitionally control [DA]ex was not extinguished by one day without reward. The same effect that occurred on day 4 occurred again on day 6 when feedback was once again omitted (Foo et al., 2021).
The increased amplitude and duration of spontaneous dopamine impulses in the mice provided with neurofeedback in the form of a reward suggests that the mice were able to learn to volitionally control [DA]ex independently of sensory stimuli (Foo et al., 2021). This finding could be significant for advances in neuromodulation therapy in humans, which is the control of neural activity using targeted stimulus delivery (International Neuromodulation Society, 2014). Neuromodulation therapy is used to treat brain disorders such as chronic neuropathic pain, Parkinson’s disease, and epilepsy, or mental illnesses such as depression and obsessive-compulsive disorder (International Neuromodulation Society, 2014). In major depressive disorder (MDD) in particular, the dysregulation of afferent dopaminergic neurons (those releasing extrasynaptic dopamine) is thought to be one of the main pathophysiological mechanisms (Belujon & Grace, 2017). Several studies have investigated the use of neurofeedback for treatment of MDD, specifically monitoring brain wave patterns (using electroencephalogram, or EEG) or dopaminergic signaling (using real-time functional magnetic resonance imaging, or rtfMRI) (Lee et al., 2019; Cheon et al., 2016; Trambaiolli et al., 2021; Sulzer et al., 2013). Foo and colleagues’ finding that spontaneous dopamine impulses can be volitionally controlled using neurofeedback may further reinforce the promise of neuromodulation therapies for MDD and other brain disorders featuring dopaminergic dysregulation (2021).
References
- Georgia Institute of Technology. (2021, July 21). Wearable brain-machine interface turns intentions into actions: New system based on user’s motor-imagery could control wheelchair, robotic arm, or other devices. ScienceDaily. Retrieved August 26, 2021 from www.sciencedaily.com/releases/2021/07/210721120657.htm
- Hcomiskey@himss.org. (2021, June 4). The endless possibilities of wearable technology in healthcare. HIMSS. https://www.himss.org/resources/endless-possibilities-wearable-technology-healthcare.
- Marr, B. (2021, March 19). The biggest wearable technology trends in 2021. Forbes. https://www.forbes.com/sites/bernardmarr/2021/03/05/the-biggest-wearable-technology-trends-in-2021/?sh=4f4b87b33092.
- Studio, P. (2016). Approach. Neuralink. https://neuralink.com/approach/.
- Wang, X.-J., Hu, H., Huang, C., Kennedy, H., Li, C. T., Logothetis, N., Lu, Z.-L., Luo, Q., Poo, M.-ming, Tsao, D., Wu, S., Wu, Z., Zhang, X., & Zhou, D. (2020, June 12). Computational neuroscience: A frontier of the 21st century. OUP Academic. https://academic.oup.com/nsr/article/7/9/1418/5856589.
Related Posts
Are There ‘Supertaskers’ Among Us?
Figure 1: Shows a graphic of an animated, and relaxed...
Read MoreVideo Games Shape Childhood Cognitive Development
Source: Caleb Oquendo The first video game was introduced in...
Read MoreBile Acid-Induced Satiety to Treat Obesity
Figure 1: Diagram of the effects of bile acids on...
Read MoreMind Missing Out? Fear of Missing Out and the Brain
Figure: A person scrolling through news articles on their smartphone....
Read MoreStrength in Numbers: How Community Enhances Perceived Support
Figure 1: Bandmates join in a group hug. The powerful...
Read MoreKrithika Nathamuni