Cover Image: Grand Prismatic Spring in Yellowstone National Park (Source: Clément Bardot, Wikimedia Commons)
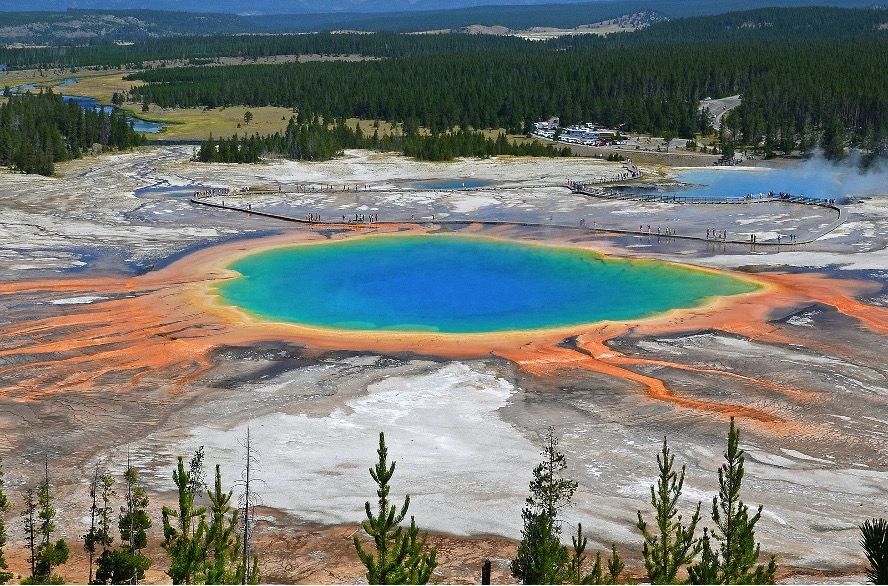
Introduction
Yellowstone National Park was the world’s first national park, designated as such by President Ulysses S. Grant in 1872 because of its well renowned hydrothermal properties and geologic wonders (U.S. Department of the Interior, 2021; U.S. Department of the Interior, 2020b). The park contains the world’s most concentrated area of geysers, hot springs, mudpots, and steam vents, with over 10,000 in total. The country’s largest and most photographed spring, the Grand Prismatic Spring shown in Figure 1, is located in the Midway Geyser Basin of the park. The spring is over 10-stories deep, 370 feet in diameter, and contains a rainbow of colors produced by the thermophilic bacteria that call the spring home (Peglar, 2017). Yellowstone National Park is geographically divided into geyser basins, defined as locations with clusters of hydrothermal features. Hot springs are the most common hydrothermal features in Yellowstone, but basins can also include geysers, mudpots, and fumaroles.
The park’s thermophilic features are ideal locations to explore the fundamental questions regarding life under extreme conditions. Prior research on heat-resistant microbes has led to both medical and forensic scientific advancements, such as the polymerase chain reaction (PCR) – which has proved an extremely valuable tool for gaining information on poorly understood diseases. Life within the park has also provided insight into Earth’s past microbial communities, and it has even helped researchers develop proposals about life on other planets.
Hot Spring Formation and the Grand Prismatic Spring
Hot springs are formed when water from deep within the earth emerges through the cracks in its surface (Geiling, 2016). In this case, the internal water route does not contain any obstructions near the surface that are responsible for creating intense, sporadic eruptions, as seen in geysers. In contrast, the hot water that comes out of hot springs is able to continuously flow and create a nonstop cycle of hot water rising, cooling, and falling.
Tourists in Yellowstone National Park often take pictures of the beautiful hot springs, capturing the rainbow coloration provided by the spring’s large groupings of microbial life (U.S. Department of the Interior, 2019b). The different thermophilic microbes present within the spring all have their own desired temperatures, and they cannot tolerate much cooler or warmer conditions. Hot springs have a distinct color gradation where the temperature of one microbial group is reached and another microbial community begins as the water temperature changes. This doesn’t mean that all colors are produced by single microbial species – often the communities within the colored mats are mixtures of several different microbes – but depending on the temperature, some organisms will be more predominant than others (Geiling, 2016).
The outermost ring of bacteria in the Grand Prismatic Spring, Calothrix, is responsible for the dark brown mats that are seen in Figures 1 and 2. The bacteria thrives in temperatures between 86 and 113 degrees Fahrenheit (U.S. Department of the Interior, 2020a). Moving inward towards the region heated to a range of 95 and 135 degrees Fahrenheit, Phormidium is the predominant creator of the orange mat color. Calothrix and Phormidium are both bacterial genera within the phylum cyanobacteria, meaning that they derive their energy from the sun through photosynthesis. Further inward, the species Aquifex Hydrogenobaclum lives at a comfortable 131 to 162 degrees Fahrenheit, and it is responsible for the spring’s yellow and white colors. Although present at the Grand Prismatic Spring, it is found more abundantly in Yellowstone’s Norris Geyser Basins and Amphitheater Springs. Its metabolic pathways consist of using hydrogen, hydrogen sulfide, and carbon dioxide as energy sources, but it can also use arsenic in place of hydrogen sulfide if necessary.
The green and yellow mats are both created by the genus Synechococcus, which lives at temperatures between 126 to 165 degrees Fahrenheit and can actually produce a range of different colors. Synechococcus starts to be seen as yellow when the temperature exceeds 149 degrees (Geiling, 2016). That is the point in which the carotenoid pigment within its chloroplasts becomes more predominant than its chlorophyll pigments. Synechococcus, like Calothrix and Phormidium, are genera of the phylum cyanobacteria, and undergo photosynthesis. The primary pigment for photosynthesis (the conversion of energy from the sun into chemical energy channeled by molecules within the bacteria) is chlorophyll, which we see as green, but chlorophyll levels can become surpassed by carotenoid pigments in more extreme conditions, which can be seen as red, orange, or yellow. The Synechococcus genus inhabiting the yellow temperature band live under harsher conditions and produce more carotenoids than they would if they lived under optimal temperature conditions such as 64 and 69 degrees Fahrenheit (Mackey et al., 2013). Synechococcus living in less harsh sunlight would look more blue-green – and that is how it is commonly seen in most lakes and ponds elsewhere (Geiling, 2016). The carotenoids act like sunscreen to protect the bacteria from extreme sunlight as high-energy ultraviolet wavelengths are captured.
It’s important to keep in mind that each color of the Grand Prismatic Spring does not represent a distinct bacteria but is a composite color that represents a community of organisms, inhabiting a desired temperature range. One example of a bacteria that is found alongside a number of others in a very broad temperature ranges is the green non-sulfur metabolizing Chloroflexus, which lives throughout the 95 to 185 degree range. It is the only microbe present in the hot spring capable of anoxygenic photosynthesis – photosynthesis that produces no oxygen (U.S. Department of the Interior, 2020a).
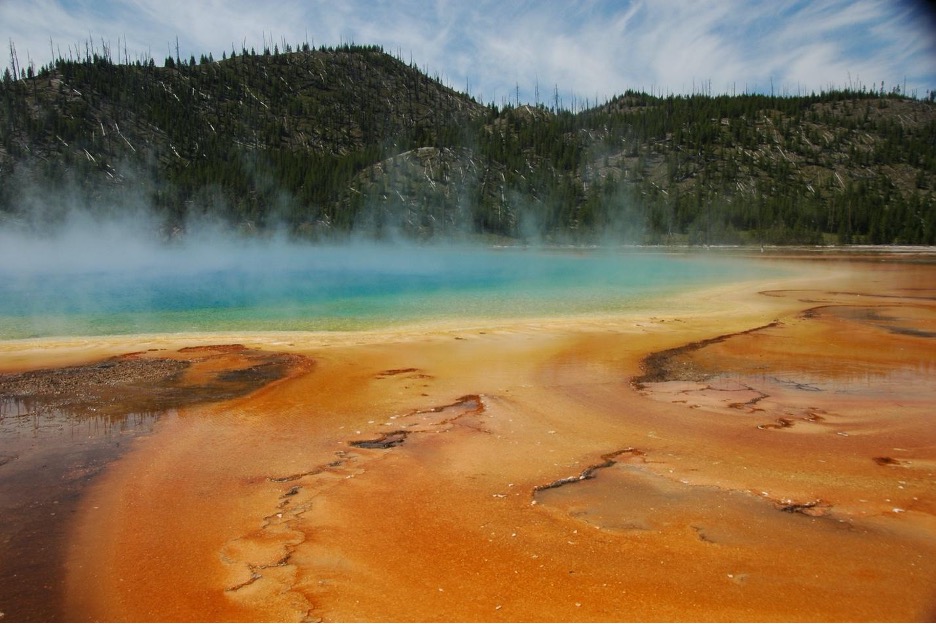
Figure 2: A closer look at Calothrix, responsible for the Grand Prismatic Spring’s brown bacterial mats, as well as the orange mats that are primarily derived from Phormidium. (Source: James St. John, Flickr)
The Grand Prismatic Spring’s beautiful rainbow configuration is mostly derived from the bacterial phylum cyanobacteria, which includes the individual genera Calothrix, Phormidium, and Synechococcus. Cyanobacteria have been responsible for more than just creating colorful pigments for tourists to gawk at, however. They have also shaped the course of evolution and ecological change throughout earth’s history due to their ability to perform oxygenic photosynthesis, which has led to the oxygen-rich atmosphere that we have today (Introduction to the Cyanobacteria, n.d.). Because cyanobacteria can live in both anerobic and aerobic conditions, it is believed that they were responsible for the initial rise of atmospheric oxygen (Kasting & Siefert, 2002). Now, the atmosphere is comprised of 21% oxygen but before the great oxidation event, the time period when Earth’s atmosphere first experienced an increase in oxygen during the Paleoproterozoic era, the chemistry of our atmosphere was vastly different and unsuitable for the life present today (National Geographic Society, 2019). It was comprised mainly of gas expelled from volcanos which included hydrogen sulfide, methane, and carbon dioxide (Smithsonian Institution, n.d.).
Another contribution of cyanobacteria to our present day ecosystem can be found in the evolutionary history of plants. Plant chloroplasts – the internal structures responsible for photosynthesis – are actually believed to be the descendants of cyanobacteria. During the late Proterozoic or early Cambrian time period (scientific estimates vary), cyanobacteria began living within certain eukaryote cells, providing energy sources for the eukaryotic host in return for a safe place to live (a relationship known as endosymbiosis). Building on this theory, the hot spring’s gradient of extremophilic bacterial communities could hold various hidden secrets about the evolution of life.
Microbiologist Thomas Brock, who studied the phylum cyanobacteria, was interested in just that question. However, when he went to examine the bacteria within Yellowstone National Park, he discovered another microbe that would later transform the scientific research industry and the field of diagnostic medicine.
The Discovery of Taq DNA Polymerase
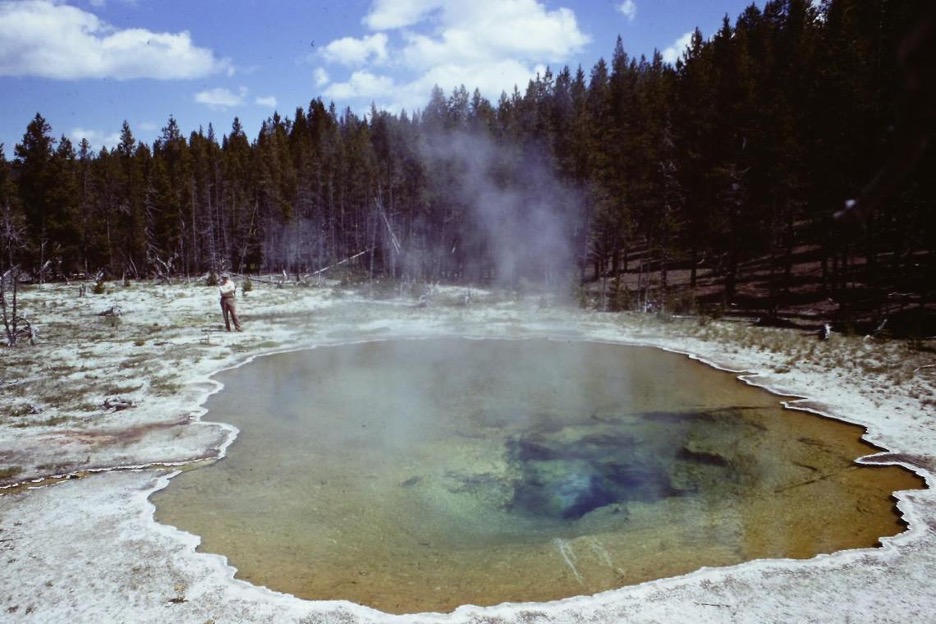
Figure 3: Mushroom Pool, located in Yellowstone National Park’s Lower Geyser Basin. Thomas Brock, seen standing near the edge, took a sample that contained T. aquaticus strain YT-1. Image from “A Scientist in Yellowstone National Park” (Brock, 2017) (Source: Thomas Brock. Public Domain sourced from USGS)
Thomas Brock began his research into the hot and acidic springs of Yellowstone in the 1960s and 70s when he was a professor of Microbiology at Indiana University. Since then, his findings have propelled modern molecular biology forward to an immense degree (Hamilton, 2017). The central focus of Brock’s research team was the isolation of unique microbes capable of eating sulfur and growing in hot acid. But Brock’s most historic accomplishment was the discovery of Thermus aquaticus, which lives comfortability at over 160 degrees Fahrenheit. Taq polymerase, shown in Figure 3, is the bacteria’s heat-resistant DNA replication enzyme, which is now being used all the time by scientists to carry out experiments that rely on a technique called polymerase chain reaction (PCR). Taq polymerase is used because it can resist the high temperatures that are required during PCR cycles. The purpose of PCR is to amplify desired DNA strands to facilitate gene sequencing, disease diagnosis, and forensic DNA identification (Hamilton, 2017).
Thomas Brock isolated T. aquaticus from a Yellowstone hot spring in the 1960s, and Taq polymerase was named Science magazine’s first-ever “Molecule of the Year” in 1989. Taq polymerase has made studying DNA practical, effective, and affordable (U.S. Department of the Interior, 2019a). “When I started in Yellowstone, I was just looking for a nice simple ecosystem where I could study microbial ecology,” explains Brock, an emeritus professor of bacteriology at the University of Wisconsin–Madison. “At higher temperatures, you don’t have the complications of having animals that eat all the microbes” (Hamilton, 2017). This discovery – in addition to revolutionizing the field of genomics – jump-started interest in thermophilic life (a ‘thermophile’ is, translated literally, a lover of extreme conditions). By 1977, the tree of life was being rewritten due to the recent discovery of extremophilic archaea, and that same year, deep-sea hydrothermal vents were considered to be the progenitors of life itself after an extremophilic discovery there as well. Thus, Thomas Brock’s research in Yellowstone ended up challenging present assumptions about nature and the resilience of life itself.
Later, Brock expanded his sampling locations to include thermal pools in California and other global sites. He was able to isolate T. aquaticus in various places besides Yellowstone National Park (Shea, 2018). His success in doing so contradicted previous scientific work that suggested that such extreme environments were not capable of supporting life. Brock’s emphasized this point in his 1967 paper, published in the journal Science and based on his work in Yellowstone, stating that “it is thus impossible to conclude that there is any ‘upper temperature of life.’” His freeze-dried sample of T. aquaticus from Yellowstone’s Mushroom Pool remains today at the American Type Culture Collection of the American Microbiology Society.
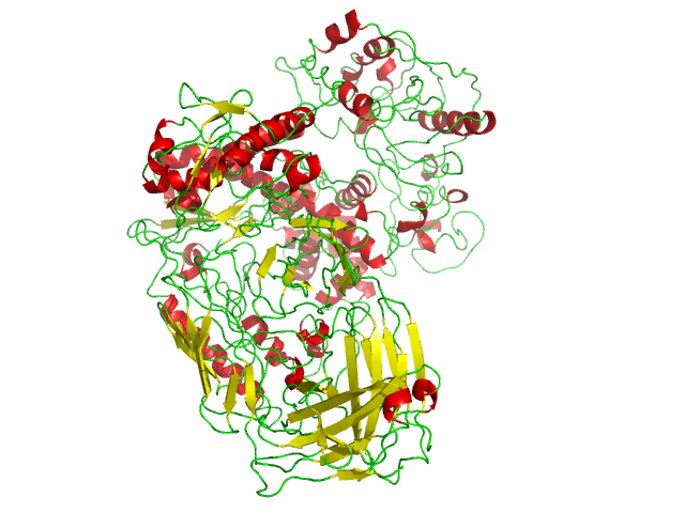
Figure 4: Taq polymerase enzyme, the 1989 Science magazine’s “Molecule of the Year” (Source: Lijealso, Wikimedia Commons)
Mammoth Hot Springs
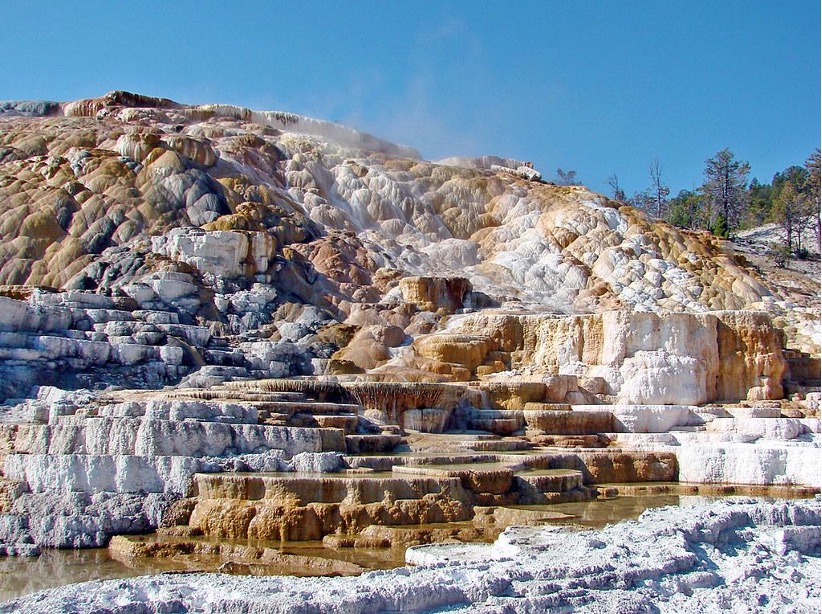
Figure 5: Mammoth Hot Springs in Yellowstone National Park, Wyoming, USA (Source: Don Graham, Flickr)
Another thermophilic feature of Yellowstone National Park is the Mammoth Hot Springs. Water in the springs trickles over each platform’s edge to create the effect of a frozen waterfall. The cyanobacteria genus Oscillatoria produces the orange mats seen on the Mammoth Hot Springs where the water temperature is between 96 to 113 degrees Fahrenheit (U.S. Department of the Interior, 2020a). The bacteria derives energy from photosynthesis, and it is capable of phototaxis, the ability to move closer to or further away from a light source.
Green Sulfur Chlorobium form a dense, dark green layer. The bacteria has an ideal pH range of 7-9, and lives between 90 to 126 degrees Fahrenheit. Its metabolism consists of anoxygenic photosynthesis which releases sulfate and sulfur into the environment, instead of oxygen. At 126 degrees, the highest temperature that Green Sulfur Chlorobium is able to withstand, begins the ideal temperature threshold for the cyanobacteria genus synechococcus, which ends at 165 degrees. Synechococcus is capable of performing photosynthesis by day and fermentation by night (U.S. Department of the Interior, 2020a).
While the intricate fountains of the Mammoth Hot Springs are home to several thermophilic microorganisms, its pools could help us discover life on other planets. The rapid deposition of minerals in the Springs traps thermophilic communities, which serve as an environmentally sensitive fossil record (U.S. Department of the Interior, 2019c). The primary microbe that is being entombed is the bacterial genus Sulfurihydrogenibium (Dong et al., 2019). Lipids from the bacteria can also become trapped within the springs and preserved in a solid-phase. Their extraction may provide key evidence in the reconstruction of ancient microbial communities (Fouke, 2011). Researchers have drawn parallels between the harsh living environments on earth and applied the concept to the possibility of life on other planets.
New research conducted at the University of Illinois has shown that the bacterium Sulfurihydrogenibium yellowstonense thrives in harsh living conditions that are similar to those expected on Mars (Yates, 2019). Their S. yellowstonense sample was sourced from the Mammoth Hot Springs. The bacterium belongs to a lineage that evolved before Earth’s oxygenation, approximately 2.35 billion years ago. It is capable of survival in extremely hot, fast-flowing water, and even ultraviolet exposure. The microbe requires extremely low oxygen levels, and derives its energy from utilizing sulfur and carbon dioxide. University of Illinois geology professor Bruce Fouke, leader of the NASA-funded study, stated that, “taken together, these traits make it a prime candidate for colonizing Mars and other planets.” The proteins present on the bacteria’s surface are capable of speeding up crystallization of calcium carbonate, resulting in the deposition of broad slash-like marks of hardened rock with a filamentous texture. Consequently, the microbe’s physical appearance resembles fettuccine pasta. Fouke also stated that, “if we see the deposition of this kind of extensive filamentous rock on other planets, we would know it’s a fingerprint of life” (Yates, 2019).
Connections can be drawn between the thermophilic conditions of Yellowstone National Park that contain life and the possibility of life on other plants. Yellowstone’s hydrothermal features contain examples of earth’s earliest forms of life, exhibiting both chemosynthesis and photosynthesis metabolism, like a window into Earth’s ancient past (U.S. Department of the Interior, 2019c). The chemosynthetic microbes that inhabit some of Yellowstone’s hot springs metabolize inorganic chemicals, a process that does not require the usage of sunlight like photosynthesis. Microbial life that thrives through chemosynthesis is more likely to occupy extra-terrestrial environments such as Mars or the moons of Jupiter than microbes that rely on sunlight. Microbial communities within the park demonstrate the extreme conditions that life is capable of enduring and provide clues to diverse environments that could harbor life on other worlds, or even unique locations within our own world like the hydrothermal vents deep within the ocean floor.
Conclusion
Yellowstone National Park held the secrets of genetic sequencing within T. aquaticus and the mysteries of life on other planets within Sulfurihydrogenibium yellowstonense. With numerous other communities of thermophilic life within its hot springs and mud pots, the park holds so much more to discover. Thomas Brock once stated that “Yellowstone really was a lark, like Let’s go look at these hot springs for fun. And what starts as intellectual enjoyment becomes a full-blown project” (Hamilton, 2021). Indeed, scientific research at Yellowstone is still very much a full-brown project. This location became protected as the world’s first national park because of its hydrothermal wonders, and it remains protected still for the benefits and curiosities its heat-resistant microbial life could bring us.
References
Dong, Y., Sanford, R. A., Inskeep, W. P., Srivastava, V., Bulone, V., Fields, C. J., Yau, P. M., Sivaguru, M., Ahrén, D., Fouke, K. W., Weber, J., Werth, C. R., Cann, I. K., Keating, K. M., Khetani, R. S., Hernandez, A. G., Wright, C., Band, M., Imai, B. S., Fried, G. A., … Fouke, B. W. (2019). Physiology, Metabolism, and Fossilization of Hot-Spring Filamentous Microbial Mats. Astrobiology, 19(12), 1442–1458. https://doi.org/10.1089/ast.2018.1965
Fouke, B. W. (2011). Hot‐spring Systems Geobiology: abiotic and biotic influences on travertine formation at Mammoth Hot Springs, Yellowstone National Park, USA. Wiley Online Library. https://onlinelibrary.wiley.com/doi/full/10.1111/j.1365-3091.2010.01209.x.
Geiling, N. (2016). The Science Behind Yellowstone’s Rainbow Hot Spring. Smithsonian.com. https://www.smithsonianmag.com/travel/science-behind-yellowstones-rainbow-hot-spring-180950483/.
Hamilton, E. (2017). New book gives personal account of pioneering Yellowstone research. News. https://news.wisc.edu/new-book-gives-personal-account-of-pioneering-yellowstone-research/.
Hamilton, E. (2021). Tom Brock, who discovered world-changing extremophiles, dies at 94. News. https://news.wisc.edu/tom-brock-who-discovered-world-changing-extremophiles-dies-at-94/.
Introduction to the Cyanobacteria. (n.d.). https://ucmp.berkeley.edu/bacteria/cyanointro.html.
John, J. (2011). Grand Prismatic Spring (11 August 2011) [Photograph]. https://www.flickr.com/photos/jsjgeology/14299134840/in/photostream/
Kasting, F., Siefert, L. (2002). Life and the evolution of Earth’s atmosphere. Science (New York, N.Y.), 296(5570), 1066–1068. https://doi.org/10.1126/science.1071184
Mackey, K. R. M., Paytan, A., Caldeira, K., Grossman, A. R., Moran, D., McIlvin, M., & Saito, M. A. (2013, October). Effect of temperature on photosynthesis and growth in Marine Synechococcus SPP. Plant Physiology. Retrieved October 20, 2021, from https://www.ncbi.nlm.nih.gov/pmc/articles/PMC3793060/.
National Geographic Society. (2019). Atmosphere. National Geographic Society. Retrieved September 24, 2021, from https://www.nationalgeographic.org/encyclopedia/atmosphere/.
Peglar, T. (2017). Grand Prismatic Spring at Yellowstone’s Midway Geyser Basin. Yellowstone National Park Trips. https://www.yellowstonepark.com/things-to-do/geysers-hot-springs/grand-prismatic-midway-geyser-basin/.
Shea, M. (2018). Discovering Life in Yellowstone Where Nobody Thought It Could Exist . National Parks Service. https://www.nps.gov/articles/thermophile-yell.htm.
Smithsonian Institution. (n.d.). The Earliest Atmosphere. Smithsonian Environmental Research Center. https://forces.si.edu/atmosphere/02_01_00.html.
U.S. Department of the Interior. (2019a). Bioprospecting. National Parks Service. https://www.nps.gov/yell/learn/nature/bioprospecting.htm.
U.S. Department of the Interior. (2019b). Hydrothermal Features. National Parks Service. https://www.nps.gov/yell/learn/nature/hydrothermal-features.htm.
U.S. Department of the Interior. (2019c). Thermophiles in Time and Space. National Parks Service. https://www.nps.gov/yell/learn/nature/thermophiles-in-time-and-space.htm.
U.S. Department of the Interior. (2020a). Thermophilic Bacteria. National Parks Service. https://www.nps.gov/yell/learn/nature/thermophilic-bacteria.htm.
U.S. Department of the Interior. (2020b). Birth of a national park. National Parks Service. Retrieved September 22, 2021, from https://www.nps.gov/yell/learn/historyculture/yellowstoneestablishment.htm.
U.S. Department of the Interior. (2021). Hydrothermal Systems. National Parks Service. https://www.nps.gov/yell/learn/nature/hydrothermal-systems.htm.
Yates, D. (2019). ‘Fettuccine’ may be most obvious sign of life on Mars, researchers report. ILLINOIS. https://news.illinois.edu/view/6367/792185.
Related Posts
How The Fight For Protection of Indigenous Land Helps The Environment
Figure 1: The sheer amount of deforestation of the Amazon...
Read MoreGeopolymer Cement: Upscaling Industrial Waste into Sustainable Alternatives to Portland Cement
Figure 1: Aerial view of the 1.1 billion gallons of...
Read MoreUncovering (Ant)imicrobial Agents in Insects
Figure 1: Insect microbiomes offer a promising source for the...
Read MoreWhen Breaking the Ice Isn’t So Simple: The Phenomenon of Flexible Ice Crystals
Figure: Icicles like these, which have formed on a house...
Read MoreTwin Impacts of the Chernobyl Disaster: Birth Defects and Mental Health
Figure 1: Damage caused to reactor 4 of the Chernobyl...
Read MoreTo safeguard Africa’s topmost predators
From Nepal’s bengal tiger to Kenya’s big Lions, conservation of...
Read MoreAnna Schwenn