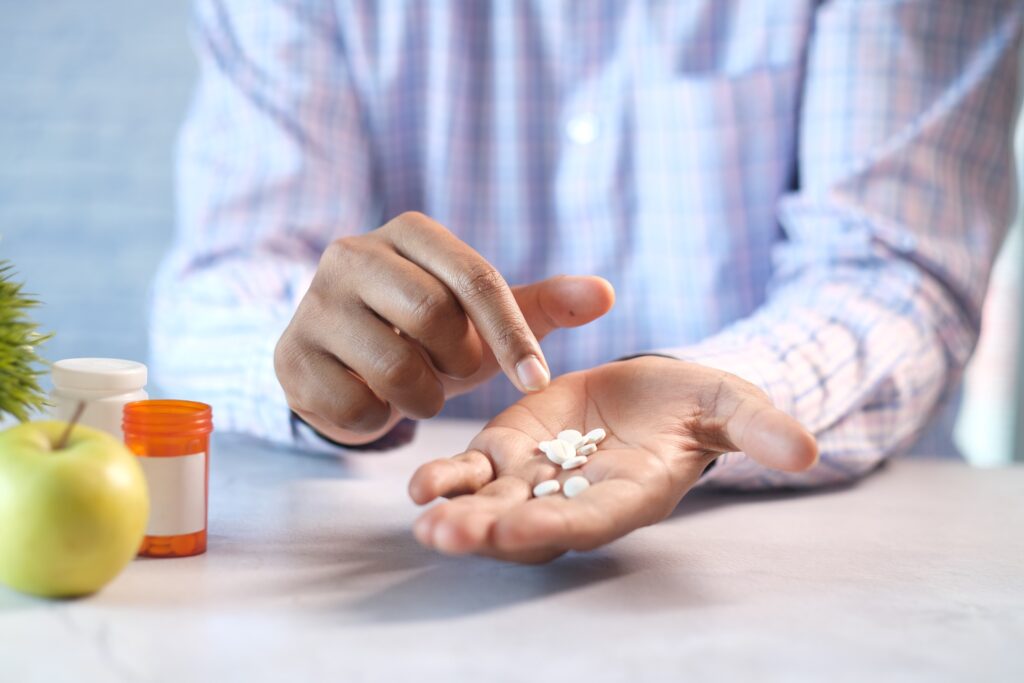
Scientists are studying the human microbiome and its ability to disable drug compounds, an occurrence which may account for drug treatment failures in people being treated for type II diabetes, cancer, and a wide range of other illnesses.
This is a complicated problem – the human microbiome is home to many diverse strains of bacteria and other microorganisms, each with their own enzymes that alter or degrade drugs. Finding the exact bacterial strain and enzyme responsible for disactivating a specific drug is like finding a needle in a haystack.
Often researchers start with what is known. For example, the bacterial strain E. lenta SM2243 has been shown in prior studies to disactivate the cardiac drug digoxin. The question is what enzymes, among the thousands of proteins in the E. lenta genome, are responsible. To zero in on the culprit, researchers exposed E. lenta SM2243 to digoxin and found that two genes (cgr1 and cgr2) became much more active after digoxin exposure, suggesting that they are being turned on to deactivate the drug. Further examination of two other E. lenta strains – neither capable of disactivating digoxin – showed that they did not possess cgr1 and cgr2, providing additional evidence that these two genes are responsible for digoxin deactivation1.
Why is it that bacteria disable human drugs? Many drug compounds are initially derived from bacteria. This includes not only antibiotics (which bacteria produce to fight off other bacteria), but also compounds with anti-cancer properties or the capacity to treat other diseases like diabetes2,3. Bacterial cells have enzymes to degrade these compounds so that they don’t harm the bacteria itself – good for the bacteria, but bad for someone receiving drug treatment. The problem gets even worse. The activity of microbial enzymes often extends to drug compounds that were not derived from bacteria to begin with. For example, the gut microbiome modifies the cancer drug irinotecan (a derivative of the natural compound camptothecin from the Chinese ornamental tree Camptotheca acuminata) and converts it into a toxic form that can cause severe diarrhea4. Digoxin is another example, derived from the foxglove species Digitalis lanata.
Fortunately, with the power of modern computers, researchers are starting to identify bacterial enzymes with drug-disarming effects more rapidly. After the initial discovery of enzymes like cgr1 and cgr2 in a single bacterial species, it becomes possible to search for similar enzymes in other bacterial species. To do so, researchers turn to ‘genome mining’. This means using computer algorithms to read through the DNA of bacteria residing in the human gut and search for genes that are similar (the technical term is homologous) to known drug resistance genes. As an example, one team of researchers started with a gene (TyrDC) from the bacterial species L. brevis that is known to disactivate the Parkinson’s Drug L-dopa. They screened through thousands of bacterial genomes provided by the Human Microbiome Project, found ten candidate bacterial strains with genes similar to TyrDC, and showed in the lab that some E. faecalis strains were in fact capable of disactivating L-dopa. This means that for Parkinson’s patients harboring certain strains of either E. faecalis or L. brevis (or both), L-dopa treatment will likely be less successful5.
A similar study, again using a ‘genome mining’ approach, has shown that many bacteria in the human microbiome possess enzymes that can degrade the diabetic drug acarbose. Results from a clinical trial affirm that patients who tested positive these microbial enzymes (referred to as Mcks) were less responsive to acarbose treatment than those who did not3.
It is worth noting that our own human proteins, too, are capable of degrading drugs. There is a whole field of research called pharmacogenomics dedicated to determining why different people, with different genetic profiles, respond differently to drug treatment6. This research has already yielded a drug called carbidopa, which is used to prevent the problem of L-dopa deactivation in certain patients by their own human enzymes. However, in the study demonstrating L-dopa deactivation by the human microbiome, the researchers found that carbidopa was not effective against bacterial enzymes5. Ensuring drug success requires taking the microbiome into account. New forms of treatment are needed that work on the microbiome like carbidopa works on human enzymes.
The digoxin study offers a hint at how this new kind of treatment could work. The deactivation of digoxin was limited when E. lenta strains were treated with arginine. Furthermore, mice fed a high protein diet (meaning high levels of arginine) were less susceptible to drug deactivation1. This means that dietary changes could help drugs work better in certain patients. The L-dopa study presents another treatment opportunity. Here, mice colonized with E. faecalis, the L-dopa disactivating bacteria, were treated with a compound called AFMT, which effectively inhibited the drug-deactivating microbial enzymes5. AFMT and carbidopa could be used in combination to provide extra assurance that L-dopa treatment will work. Collectively, these new forms of therapy will ensure that the power of life-preserving drugs are not stymied by the microbiome.
References
- Haiser, H. J. et al. Predicting and manipulating cardiac drug inactivation by the human gut bacterium Eggerthella lenta. Science 341, 295–298 (2013).
- SITNFlash. Bacteria: The drug factory you’d never expect. Science in the News https://sitn.hms.harvard.edu/flash/2020/bacteria-the-drug-factory-youd-never-expect/ (2020).
- Balaich, J. et al. The human microbiome encodes resistance to the antidiabetic drug acarbose. Nature 600, 110–115 (2021).
- Reardon, S. Gut bacteria can stop cancer drugs from working. Nature (2017) doi:10.1038/nature.2017.22109.
- Rekdal, V. M., Bess, E. N., Bisanz, J. E., Turnbaugh, P. J. & Balskus, E. P. Discovery and inhibition of an interspecies gut bacterial pathway for Levodopa metabolism. Science 364, eaau6323 (2019).
- National Institute of General Medical Sciences. National Institute of General Medical Sciences (NIGMS) https://nigms.nih.gov/.
Related Posts
Health Literacy in the United States
This publication is in proud partnership with Project UNITY’s Catalyst...
Read MoreAre We Driving Our Dogs Sick Through Our Urban Lifestyles?
Figure 1: Allergic disorders in humans and dogs are associated...
Read MoreConcierge Medicine Could Reduce Senior Health Inequities in Illinois
This publication is in proud partnership with Project UNITY’s Catalyst Academy 2023...
Read MoreThe H.U.N.G.E.R. Initiative (Helping Underserved Neighborhoods Generate Equitable Resources)
This publication is in proud partnership with Project UNITY’s Catalyst Academy 2023...
Read MoreUnderstanding the Social Factors Affecting Cancer Therapy
Cover Image: A patient being prepared for radiation therapy. (Source:...
Read MoreA Critical Evaluation of Strategies to Reduce Intergroup Prejudice
Figure 1: People group crowd Source: Pixabay Prejudice refers to an...
Read MoreSam Neff